Researchers working at the ALS have recently made new discoveries in understanding the nature of charge storage in lithium-ion (Li-ion) batteries, opening up possibilities for new battery designs with significantly improved capacity. Looking at a popular Li-rich cathode material, the researchers used soft x-ray techniques to quantifiably explain oxygen’s role in Li-ion charge capacity.
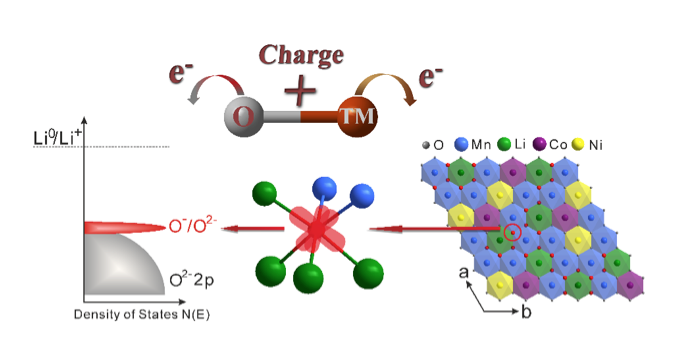
Li-ion batteries are popular power sources found in almost all portable electronics. The batteries operate by removing (deintercalating) Li+ ions from the cathode during charge and reinserting (reintercalating) them on discharge. The cathode material is key to the battery’s capacity, and is usually composed of lithium, one or more transition metals, and oxygen. Since transition metals can exist in different oxidation states with different numbers of electrons, by changing the oxidation state of the transition metal, scientists can manipulate how the electrons are stored. The charge balance is maintained by the oxidation and reduction of the transition-metal cations. Previous research had shown that Li-rich cathode materials exhibited a charge capacity that couldn’t be explained by the role of transition metals alone, but the exact reason for this had remained unclear until recently.
The role of oxygen has traditionally been assumed to be simply that of a spectator. But scientists have recently realized that oxygen can actively contribute to the battery’s storage capacity. If the transition-metal and oxygen ions could both undergo redox reactions then electrons would be stored on both, which would result in cathodes with a significantly higher energy storage. The recent ALS research has further illuminated oxygen’s role, showing that it forms localized electron holes during the charging process, which enhances the battery’s capacity.
Researchers from the University of Oxford, the University of Kent, the ALS, and Uppsala University focused on the high-capacity “Li-rich” cathode Li1.2Ni0.13Co0.13Mn0.54O2, due to its commercial relevance. Conventional techniques such as electrochemistry, mass spectroscopy, and x-ray absorption near-edge structure spectroscopy (XANES) found that transition metal redox could not explain the amount of charge stored, nor could oxygen loss in the form of gas. Therefore, the researchers looked for further understanding within the electronic structure of the oxygen.
To examine the oxygen’s electronic structure more deeply, the researchers used soft x-ray absorption spectroscopy (SXAS) and resonant inelastic x-ray scattering (RIXS) at ALS Beamline 8.0.1 to observe as the battery was charged and discharged. Soft x-rays are one of the few tools that can reveal details about the electronic structure surrounding oxygen in solid materials. The technique effectively allowed the researchers to detect the number of electron hole states associated with the oxygen and with the transition metal. Since the researchers already had a picture of the changes occurring on the metal from their XANES studies, they could conclude that the balance must be electron hole states on the oxygen. A series of samples that were removed from batteries at a variety of states of charge were studied at Beamline 8.0.1.
The researchers found that when the battery was fully charged, oxide ions with localized electron holes were formed and were surrounded by Mn4+/Li+, which interact with oxygen less covalently and therefore are located at the top of the O 2p band. This phenomenon has significant implications for charge storage, as battery materials have traditionally been limited to redox changes on transition metal ions. Understanding the activation of oxygen redox has the potential to break this limit and give scientists the tools to design new compounds with capacities in excess of what was previously thought possible.
Because of the high-quality instruments at ALS beamlines, for the first time researchers were able to show direct quantifiable evidence of the formation of localized electron holes on oxygen. Now that it is clear that they exist, scientists can do more research to better understand the phenomenon.
Understanding this material could result in the discovery of new materials with higher capacities and ultimately could result in mobile phones that last longer between charges or electric vehicles that have a much longer driving range.
Contact: Matthew Roberts
Research conducted by: K. Luo, M.R. Roberts, R. Hao, N. Guerrini, and P.G. Bruce (University of Oxford); D.M. Pickup, A.V. Chadwick (University of Kent); Y-S. Liu, J.-H. Guo (Advanced Light Source); and K. Edström, L.C. Duda (Uppsala University).
Research funding: U.K. Engineering and Physical Sciences Research Council. Operation of the ALS is supported by the U.S. Department of Energy, Office of Basic Energy Sciences.
Publication about this research: K. Luo, M.R. Roberts, R. Hao, N. Guerrini, D.M. Pickup, Y-S. Liu, K. Edström, J.-H. Guo, A.V. Chadwick, L.C. Duda, and P.G. Bruce, “Charge-compensation in 3d-transition-metal-oxide intercalation cathodes through the generation of localized electron holes on oxygen,” Nature Chemistry 8, 684 (2016).
ALS SCIENCE HIGHLIGHT #334