SCIENTIFIC ACHIEVEMENT
Researchers solved the structure of a bacterial toxin bound to a neutralizing protein, revealing two distinct mechanisms for how the toxin-producing bacteria avoid poisoning themselves.
SIGNIFICANCE AND IMPACT
The findings offer clues to the evolutionary origins of the potent toxins that enable bacterial pathogens to cause human diseases such as cholera and diphtheria.
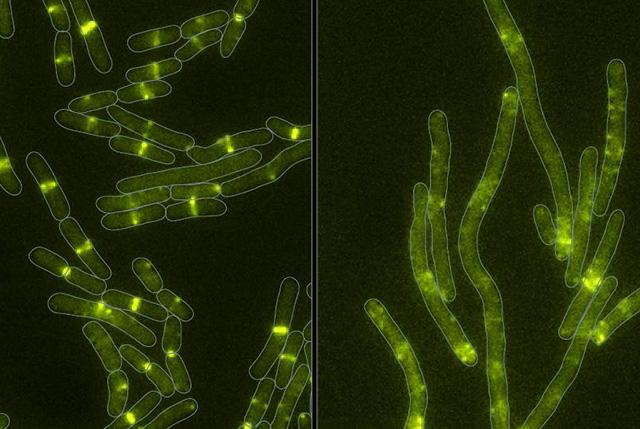
The battle for microbial domination
Microbial communities are of fundamental importance to virtually all natural ecosystems, from the ocean floor to the gastrointestinal tract. Although the term “communities” implies cooperation, scientists now realize that bacterial colonies compete with each other for life-sustaining resources, availing themselves of a variety of strategies to reduce overcrowding. In some cases, they secrete toxins in their fight for survival. Here, researchers studied one such toxin from the bacterium Serratia proteamaculans, various strains of which live inside tree roots or inhabit the digestive tracts of insects and other animals.
Toxin targets cell division
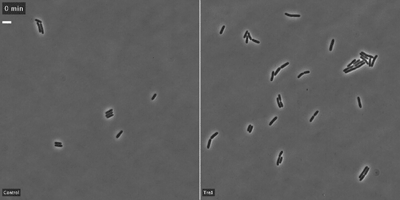
The researchers showed that the toxin, Tre1, targets a bacterial protein, FtsZ, which is analogous to tubulin in human cells. Tubulin molecules are the building blocks of microtubules—long polymers that provide structure and shape to our cells and play an important role in cell division. In bacteria, FtsZ loses the ability to polymerize when attacked by the Tre1 toxin. Instead of dividing, the intoxicated cells grow longer and longer until they eventually split open and die (cellular elongation and lysis).
Genomic analyses suggested that the toxin’s attack occurs via an enzymatic mechanism known as ART activity. Toxins that use this mechanism include those made by the pathogens responsible for human diseases such cholera and diphtheria. In addition, the researchers identified (in the same bacteria) genes for enzymes that reverse ART activity, suggesting a mechanism for bacterial immunity against self-intoxication.
Structure reveals two distinct immunity mechanisms
To gain insight into how toxin-producing bacteria counteract their own poisons, the researchers came to ALS Beamline 5.0.2, part of the Berkeley Center for Structural Biology. Using protein crystallography data collected at the beamline, they determined the structure of the bacterial toxin, Tre1, bound to its predicted antitoxin, a protein they called Tri1.
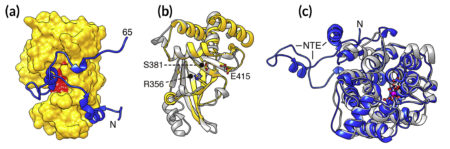
The structure revealed a striking protuberance from the main body of the antitoxin that physically blocks the toxin’s active site. In addition, comparison of the structures with those of other proteins known to produce similar enzymatic effects (i.e., ART activity and its reversal) revealed a high degree of similarity. The observations suggested that the antitoxin employs two distinct mechanisms that provide immunity: physical blocking of the toxin’s active site and reversal of its enzymatic attack mechanism.
Subsequent experiments showed that each mechanism alone is sufficient to counteract the effects of the Tre1 toxin; however, the enzymatic reversal mechanism additionally provides broad immunity to a variety of toxins, protecting the bacterium from both its own toxins and those that function by the same mechanism but are made by other species.
Evolutionary connections to human diseases
Given that encounters between bacteria vastly predate the evolution of eukaryotic (e.g., human) cells, it is conceivable, or even likely, that ART toxins that target human cells evolved from ancestral proteins that were deployed by bacteria against each other. Thus, this inside look at the chemical warfare and biodefense strategies among bacteria in their own communities can offer clues to the evolutionary origins of the potent toxins that enable bacterial pathogens to infect multicellular organisms, including people.
Contact: Joseph D. Mougous
Researchers: S.-Y. Ting, D.E. Bosch, S.M. Mangiameli, M.C. Radey, S. Huang, Y.-J. Park, K.A. Kelly, J.K. Eng, D. Veesler, P.A. Wiggins, and S. Brook Peterson (University of Washington); S.K. Filip and Y.A. Goo (Northwestern University); M. Allaire (Berkeley Lab); and J.D. Mougous (University of Washington and Howard Hughes Medical Institute).
Funding: National Institutes of Health and Howard Hughes Medical Institute. Operation of the ALS is supported by the U.S. Department of Energy, Office of Science, Basic Energy Sciences Program (DOE BES).
Publication: S.-Y. Ting, D.E. Bosch, S.M. Mangiameli, M.C. Radey, S. Huang, Y.-J. Park, K.A. Kelly, S.K. Filip, Y.A. Goo, J.K. Eng, M. Allaire, D. Veesler, P.A. Wiggins, S. Brook Peterson, and J.D. Mougous, “Bifunctional Immunity Proteins Protect Bacteria against FtsZ-Targeting ADP-Ribosylating Toxins,” Cell 175, 1380 (2018), doi:10.1016/j.cell.2018.09.037.
Adapted from the University of Washington School of Medicine news release, “With toxin, bacteria prove old dogs can learn new tricks.” See also the Berkeley Lab Biosciences story, “Newly Characterized Toxin Gives Bacterium a Leg Up on the Competition.”
ALS SCIENCE HIGHLIGHT #388