Scientists have found a way to engineer the atomic-scale chemical properties of a water-splitting catalyst for integration with a solar cell, and the result is a big boost to the stability and efficiency of artificial photosynthesis. The goal was to strike a careful balance between the contradictory needs for efficient energy conversion and chemically sensitive electronic components, in order to develop a viable system of artificial photosynthesis to generate clean fuel. The research, which involved multiple x-ray experiments performed at the ALS and the Stanford Synchrotron Radiation Lightsource (SSRL), comes out of the Joint Center for Artificial Photosynthesis (JCAP), a DOE Energy Innovation Hub established to develop a cost-effective method of turning sunlight, water, and carbon dioxide into fuel. JCAP is led by the California Institute of Technology with Berkeley Lab as a major partner.
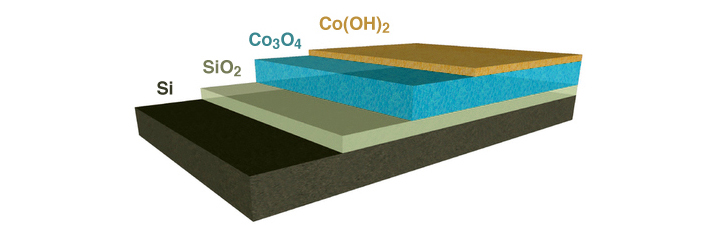
For an artificial photosystem to be viable, it should last for 20 or more years without the need for constant repair. The problem is that the active chemical environments needed for artificial photosynthesis are damaging to the semiconductors used to capture solar energy. Good protection layers are dense and chemically inactive. That is completely at odds with the characteristics of an efficient catalyst, which helps to split water to store the energy of light in chemical bonds. The most efficient catalysts tend to be permeable and easily transform from one chemical state to another. The researchers knew they needed a catalyst that, in addition to supporting active and efficient chemical reactions, would also permit as much light as possible to pass through, efficiently transfer the charge generated by the absorption of light to the catalytic sites, and provide a stable interface with the semiconductor.
The researchers turned to a manufacturing technique called plasma-enhanced atomic-layer deposition, performed at Berkeley Lab’s Molecular Foundry. This type of thin-film deposition is used in the semiconductor industry to manufacture integrated circuits and provides the level of precision needed to create the composite film. They were able to engineer a very thin layer to protect the sensitive semiconductor, then atomically join another active layer to carry out the catalytic reactions, all in a single process. The first layer of the film consisted of a nanocrystalline form of cobalt oxide, Co3O4, that provided a stable, physically robust interface with the light-absorbing semiconductor. The other layer was a chemically reactive material made of cobalt dihydroxide, Co(OH)2. The design of this composite coating was inspired by recent advances in the field that have revealed how water-splitting reactions occur, at the atomic scale, on materials.
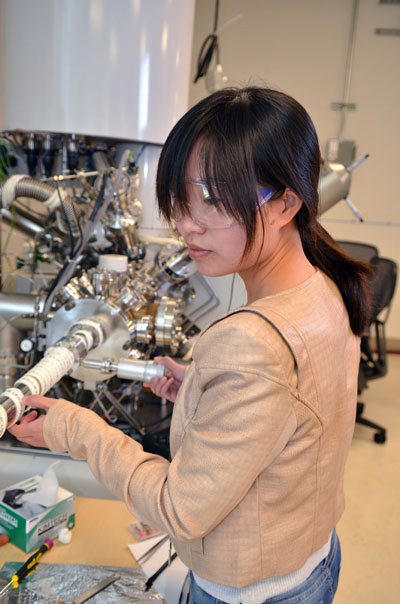
The structural and chemical properties of this new catalyst material were investigated through x-ray absorption near-edge structure (XANES) and extended x-ray absorption fine structure (EXAFS) spectroscopy experiments performed at SSRL Beamline 7.3 and soft x-ray reflectivity and scattering experiments performed at ALS Beamline 11.0.1.2. The results showed that these films can be made compact and continuous at the nanometer scale, thus minimizing parasitic light absorption when integrated on top of photoactive semiconductors. Electrochemical tests of this photosystem showed that it could run continuously for three days—potentially longer—when such systems would normally fail in mere seconds.
The study authors noted that, while this is an important milestone, many more steps are needed before a commercially viable artificial photosystem is ready for deployment. In general, they need to know more about how these systems fail so they can identify areas to target for future improvement. Understanding degradation is an important avenue to making something that is stable for decades. By engineering an atomically precise film so that it can support chemical reactions without damaging sensitive semiconductors, the researchers managed to satisfy contradictory needs for artificial photosystems.
Contact: Ian Sharp
Research conducted by: J. Yang, J.K. Cooper, F.M. Toma, K.A. Walczak, M. Favaro, J.W. Beeman, L.H. Hess, J. Yano, and I.D. Sharp (Joint Center for Artificial Photosynthesis [JCAP] and Berkeley Lab); C. Wang and C. Zhu (ALS); S. Gul, C. Kisielowski, and A. Schwartzberg (Berkeley Lab).
Research funding: Alexander von Humboldt Foundaton and the U.S. Department of Energy, Office of Basic Energy Sciences (DOE BES). Operation of the ALS is supported by DOE BES.
Publication about this research: J. Yang, J.K. Cooper, F.M. Toma, K.A. Walczak, M. Favaro, J.W. Beeman, L.H. Hess, C. Wang, C. Zhu, S. Gul, J. Yano, C. Kisielowski, A. Schwartzberg, and I.D. Sharp, “A multifunctional biphasic water splitting catalyst tailored for integration with high-performance semiconductor photoanodes,” Nat. Mater. 16, 335 (2017). doi:10.1038/nmat4794
ALS SCIENCE HIGHLIGHT #347