SCIENTIFIC ACHIEVEMENT
Researchers used the Advanced Light Source (ALS) to quantify a strong, beneficial, and reversible (over hundreds of cycles) chemical reaction involving oxygen ions in the crystal lattice of battery electrode materials.
SIGNIFICANCE AND IMPACT
The results open up new ways to explore how to pack more energy into batteries with electrodes made out of low-cost, common materials.
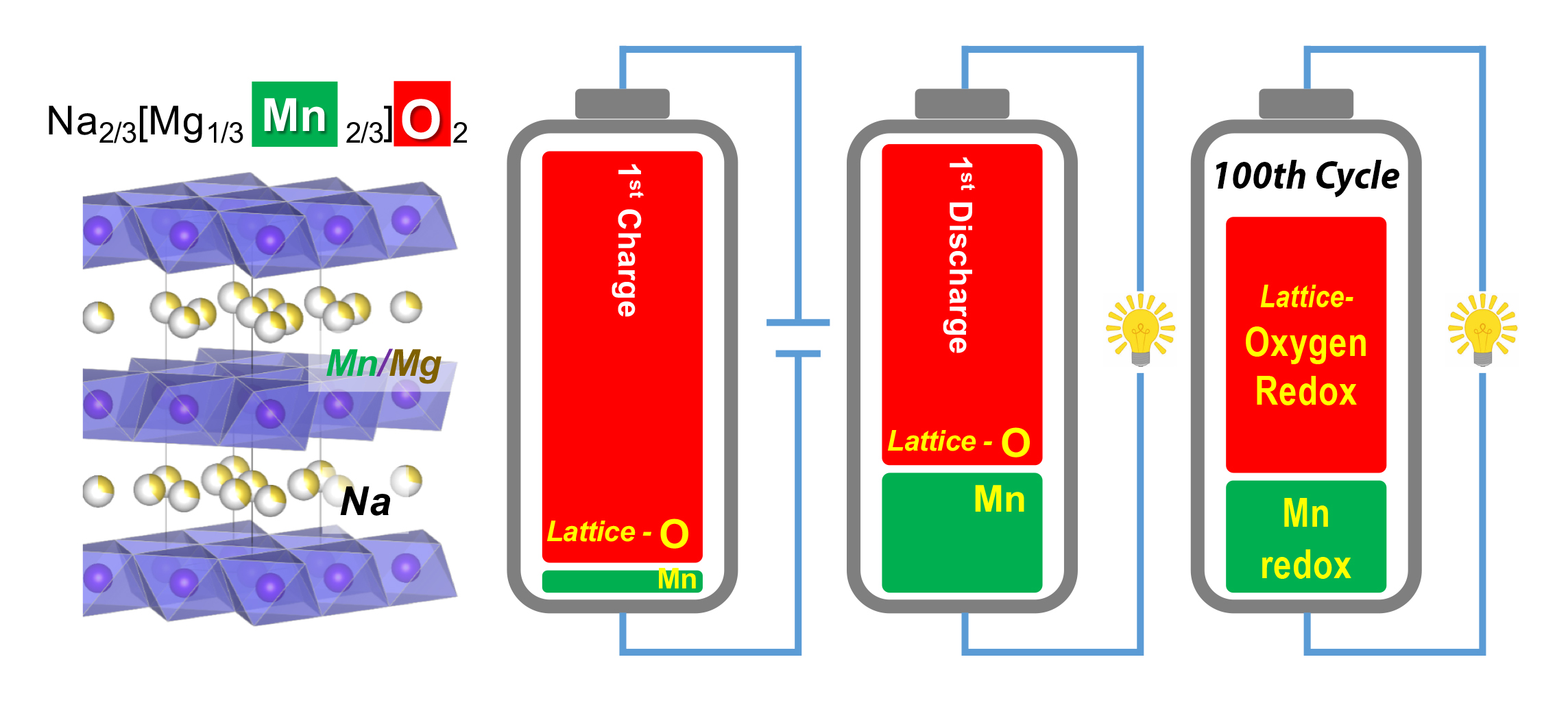
Oxygen reactions: plus or minus?
For a wide range of applications, from mobile phones to electric vehicles, the reversibility and cyclability of the chemical reactions occurring inside a rechargeable battery are key to commercial viability. Conventional wisdom had held that involving oxygen in a battery’s electrochemical operation spontaneously triggers irreversible oxygen losses and parasitic surface reactions, reducing reversibility and safety. Recently however, the idea emerged that reactions involving lattice oxygen (i.e., oxygen that’s part of the crystal-lattice structure vs oxygen on the surface) could be useful for improving battery capacity. Here, researchers report the first direct quantification of a strong, beneficial, and highly reversible chemical reaction involving lattice oxygen in electrodes made with low-cost elements.
Possibility of lattice-oxygen redox
Many rechargeable-battery electrodes are made of layers that combine oxygen with transition metals (TMs), a material class that includes relatively abundant elements such as iron, nickel, cobalt, and manganese. Oxidation-reduction (redox) reactions, which are central to battery capacity and operation, had previously been associated with these TM components. However, some TM-oxide materials demonstrated capacities far beyond what could be explained solely by TM redox, suggesting a possible contribution from the oxygen. Other studies indicated that a stable lattice-oxygen reaction would require rare and expensive TMs such as ruthenium or iridum. Although soft x-ray absorption spectroscopy helped spark these important developments, it is limited in its ability to differentiate between TM and oxygen signal contributions due to their mixed states (hybridization).
Spectral maps distinguish oxygen states
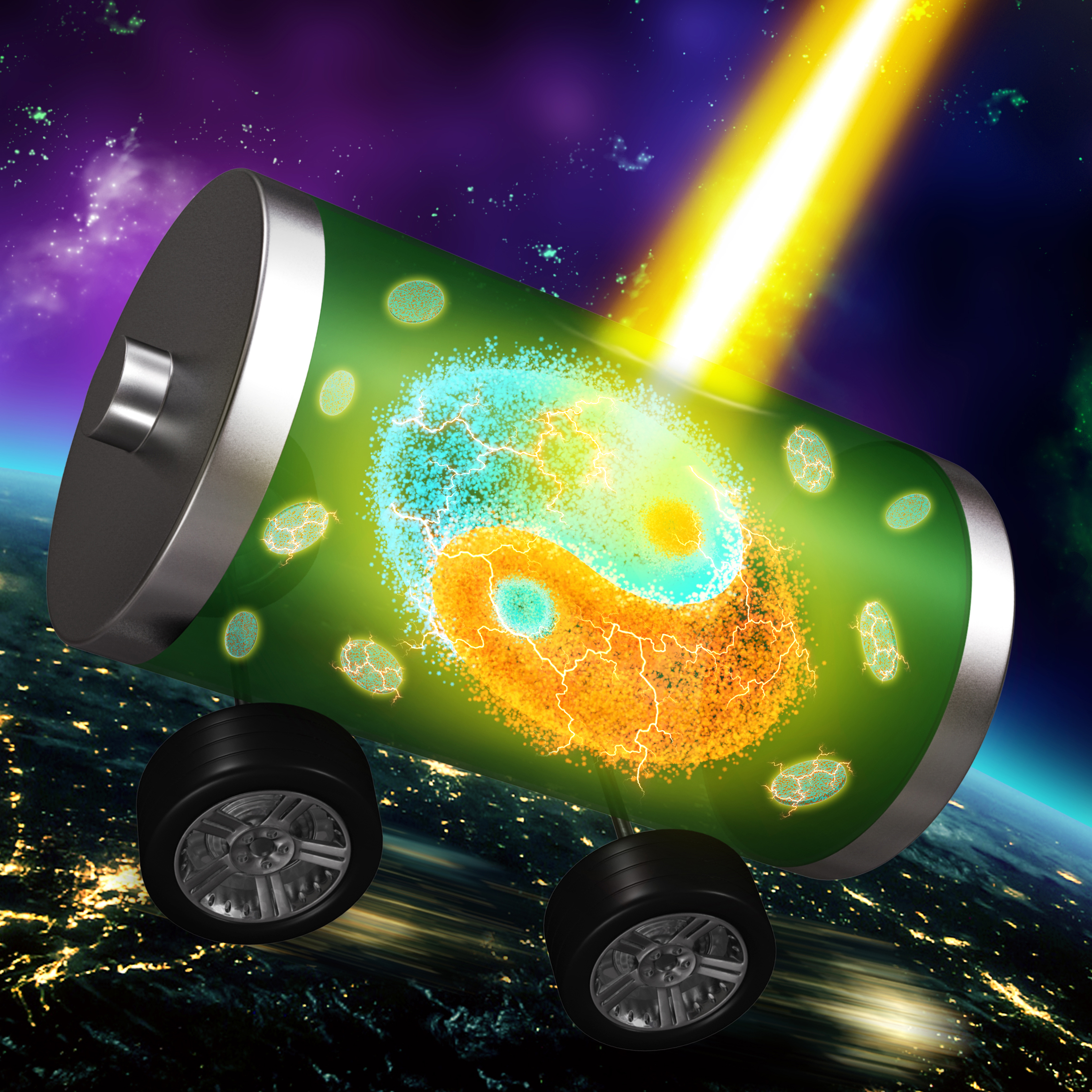
In this study, researchers fabricated and tested a sodium-ion battery material (Na2/3Mg1/3Mn2/3O2) in which manganese (Mn) is the TM. The sodium ions move in and out of the spaces between the TM-oxide layers during charging and discharging.
The samples were studied at ALS Beamline 8.0.1 using a specialized, ultrahigh-efficiency technique known as mRIXS (mapping of resonant inelastic x-ray scattering). This technique can distinguish between lattice-oxygen redox (the most useful oxygen activity) from mixed TM-oxygen redox. With mRIXS, it’s also possible to quantify reactions involving both anions (O) and cations (Mn) independently, allowing a precise understanding of the total battery capacity.
Settling the reversibility debate
The results showed that a very strong lattice-oxygen redox reaction in Na2/3Mg1/3Mn2/3O2 accounts for most of its capacity. Surprisingly, this strong reaction did not compromise reversibility: 87% of this capacity was retained after 100 charge-discharge cycles (directly seen in the mRIXS raw data). For comparison, the researchers also analyzed a more complex lithium-ion battery compound (Li1.17Ni0.21Co0.08Mn0.54O2) that exhibits other types of oxygen activity. The researchers concluded that both sodium- and lithium-ion battery materials share the same fundamental mechanism of lattice-oxygen redox that can be highly reversible for hundreds of cycles.

The study settles the debate about the reversibility of lattice-oxygen redox in battery electrodes made of common elements. It also clarifies that reductions in reversibility can be attributed to non-lattice-oxygen activities, which were often mixed with lattice-oxygen redox reactions in previous studies. Future studies could focus on different electrode materials and designs, including the use of different surface coatings and the introduction of doping agents to maximize beneficial lattice-oxygen reactions while suppressing others.
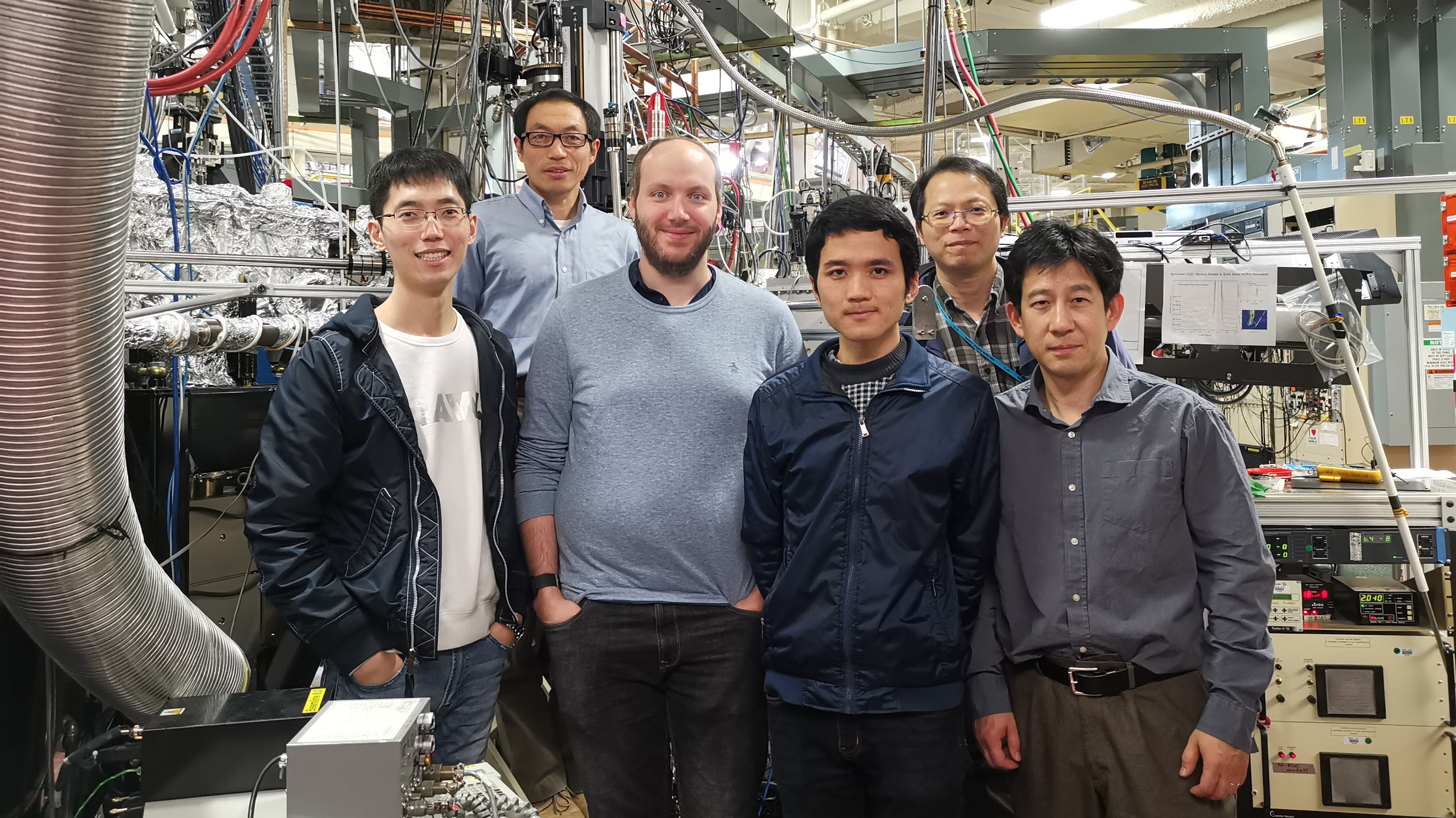
Contact: Wanli Yang
Researchers: K. Dai (Northeastern University, China, and ALS); J. Wu (Stanford University and ALS); Z. Zhuo (Peking University and ALS); Q. Li (Shandong University, China, and ALS); S. Sallis (Binghamton University and ALS); J. Mao (Zhengzhou University, China); G. Ai (Tianjin Normal University, China); C. Sun and Z. Li (Northeastern University, China); W.E. Gent and Z.-X. Shen (Stanford University); W.C. Chueh (Stanford University and SLAC National Accelerator Laboratory); Y.-D. Chuang, Z. Hussain, and W. Yang (ALS); R. Zeng (Tsinghua University, China); F. Pan (Peking University); S. Yan (Shandong University, China); L.F.J. Piper (Binghamton University); and G. Liu (Berkeley Lab).
Funding: Fundamental Research Funds for the Central Universities of China; National Natural Science Foundation of China; Science and Technology on Reliability Physics and Application of Electronic Component Laboratory open fund in China; U.S. Department of Energy (DOE), Office of Energy Efficiency & Renewable Energy; China Scholarship Council; and Energy Biosciences Institute. Operation of the ALS is supported by the DOE Office of Science, Basic Energy Sciences Program.
Publication: K. Dai, J. Wu, Z. Zhuo, Q. Li, S. Sallis, J. Mao, G. Ai, C. Sun, Z. Li, W.E. Gent, W.C. Chueh, Y.-D. Chuang, R. Zeng, Z.-X. Shen, F. Pan, S. Yan, L.F.J. Piper, Z. Hussain, G. Liu, and W. Yang, “High Reversibility of Lattice Oxygen Redox in Na-ion and Li-ion Batteries Quantified by Direct Bulk Probes of both Anionic and Cationic Redox Reactions,” Joule 3, 518 (2019), doi:10.1016/j.joule.2018.11.014.
ALS SCIENCE HIGHLIGHT #392