Throughout the summer and fall of 2014, a tragic outbreak of Ebola hemorrhagic fever in West Africa reminded us of how interconnected the world is and how vulnerable we can be to the spread of virulent diseases. Science, however, gives us powerful tools for gaining insight into how pathogens operate and, consequently, how to design strategies to defeat them. Here we report on researchers from UC San Francisco who used protein crystallography at the ALS to understand how a drug molecule that has shown some efficacy against Ebola in mice inactivates a membrane protein, called TPC1, used by viruses to infect host cells. With a high-resolution structure of the drug molecule bound to the membrane protein, pharmaceutical chemists can rationally design new drug molecules to be even more effective in shutting out these viruses.
Ebola is a member of the Filoviridae family of viruses, noted for their long, filament-like shapes. To replicate, filoviruses such as Ebola must infiltrate a host cell, using the cell’s own transport machinery against itself. The normal process of passing needed material—nutrients or metabolytes, for example—through cell membranes is called endocytosis. In this process, the membrane engulfs the material, then pinches off into a bubble (i.e., a vesicle), bringing its cargo with it. In particular, the Ebola virus must gain entry via endocytosis to certain acidic vesicles inside the cell—called endolysosomes—before its RNA can be released.
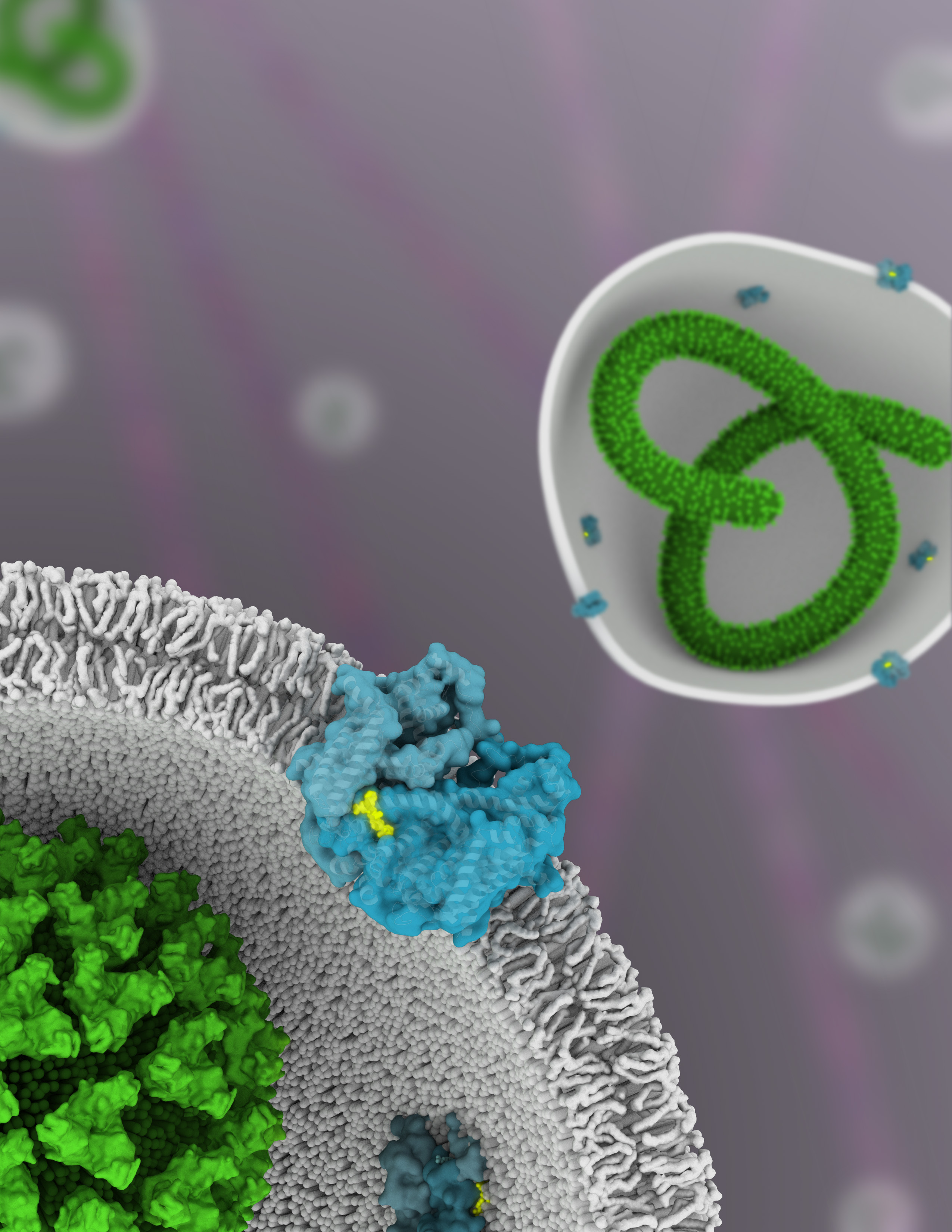
A two-pore channel (TPC) protein called TPC1, embedded in the endolysosomal membrane, is thought to govern the initial fusion between the viral and endolysosomal membranes, through signals involving the balance of calcium ions and electrical voltage across the channel. Thus, TPC1 is a voltage-gated ion channel, regulating the conductance of sodium and calcium ions through the membrane and activated by a decrease in transmembrane voltage. Calcium ions in this context are important signaling molecules: they determine how structural elements within the channel interact and how their parts get rearranged in order to, for example, intitate endocytosis with the Ebola virus.
In this work, the researchers solved the molecular structure of TPC1 to 2.87 Å using x-ray crystallography at ALS Beamlines 8.3.1 (UC Berkeley/UCSF) and 5.0.2 (Berkeley Center for Structural Biology), as well as SSRL Beamline 12-2. Co-crystallized with the TPC1 was the drug trans-Ned-19, which had previously been shown to cure 50% of Ebola-infected mice. Membrane proteins are notoriously difficult to crystallize, and their loosely packed crystals tend to diffract weakly. Thousands of crystals had to be screened by the researchers to obtain the one that ended up providing the final structure. High-brightness, high-efficiency x-ray beamlines were therefore an absolute necessity, and the beamlines’ capability for remote data collection was also extremely valuable.
The solved structure represents the first example of a voltage-sensing channel captured in the inactive “resting” state, closed off to ion flow. By comparing it with previous structures captured in the activated state, the researchers can now observe the channel’s transition from open to closed. They observed how the drug trans-Ned-19 binds to specific atoms in the channel, effectively clamping the domains together and blocking activation. They can see how charges move in the voltage-sensing domains and how those domains respond to changes in transmembrane potential. They can see how calcium signals, sensed by small folded domains on the cytosolic (internal) side of the membrane, couple to the intracellular (external) calcium concentration to open the channel. The structure shows how phosphorylation events (in which phosphate molecules are tacked onto other proteins) can open or close the channel by modifying these cytosolic domains. The data is relevant not only for Ebola treatment, but also for other diseases in which TPC1 plays a role, such as diabetes, heart disease, and neurodegenerative disorders such as Parkinson’s disease.
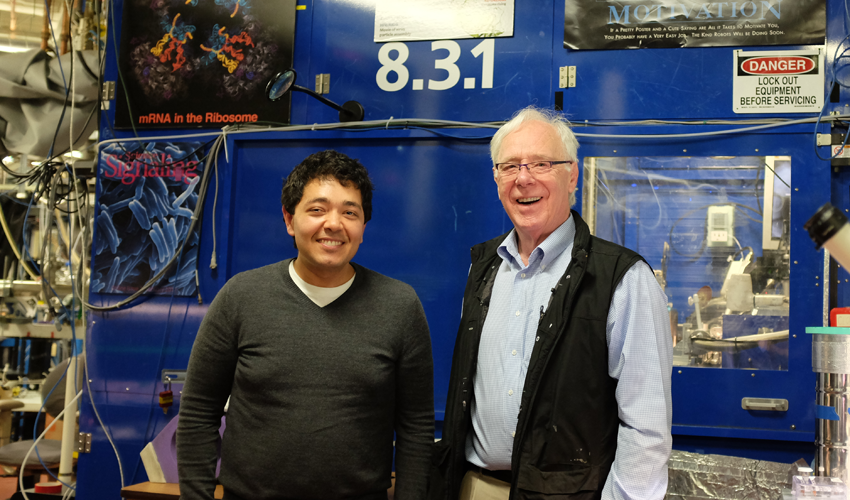
Contact: Alex Kintzer
Research conducted by: A.F. Kintzer and R.M. Stroud (Univ. of California, San Francisco).
Research funding: National Institutes of Health. Operation of the ALS is supported by the U.S. Department of Energy, Office of Basic Energy Sciences.
Publication about this research: A.F. Kintzer and R.M. Stroud, “Structure, inhibition and regulation of two-pore channel TPC1 from Arabidopsis thaliana,” Nature 531, 258 (2016).
ALS SCIENCE HIGHLIGHT #331
For previous Ebola-related studies at the ALS, see “Structure of the Ebola Virus Glycoprotein Bound to an Antibody from a Human Survivor” and “ALS Capabilities Reveal Multiple Functions of Ebola Virus.”