SCIENTIFIC ACHIEVEMENT
Spectroscopic studies done at the Advanced Light Source (ALS) clarified key reaction mechanisms in a Li-battery cathode material, revealing its surprising utility as a catalyst for next-generation batteries.
SIGNIFICANCE AND IMPACT
The work refutes widely held ideas about reversible reactions in a highly debated material for Li-based batteries and expands the range of materials suitable for use in high-power batteries and fuel cells.
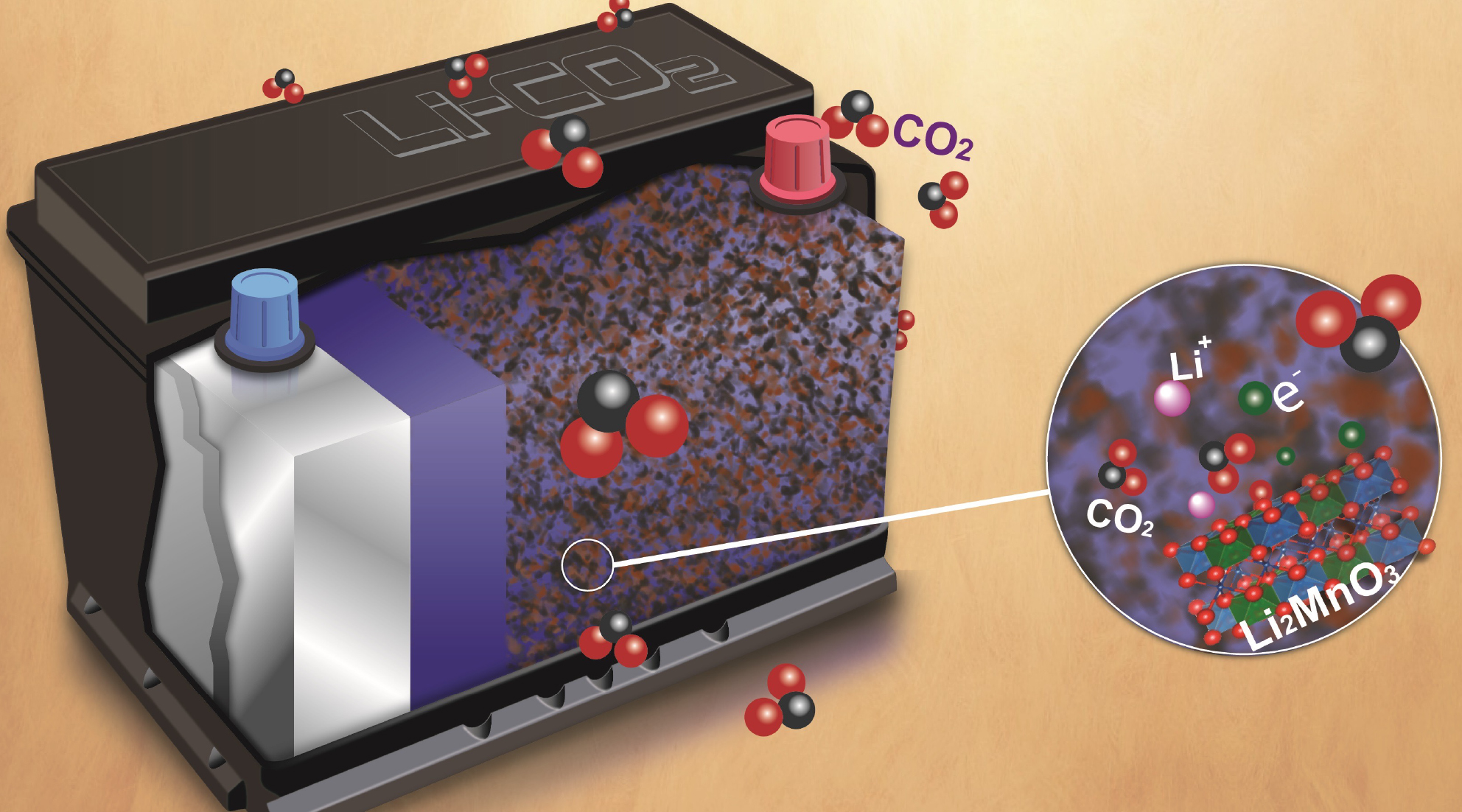
High interest in high voltage
The pressing demand for high-energy batteries capable of extending the range of electric vehicles and the operation of electronic devices has triggered tremendous efforts to develop high-voltage cathode materials for lithium-ion batteries. High-voltage operation, however, gives rise to complex chemical reactions at the cathode, involving concepts and mechanisms that are under active debate.
Through a fundamental spectroscopic study using a unique x-ray instrument at the ALS, an international team of researchers clarified the reaction mechanisms at work in a lithium-rich high-voltage cathode material. In the process, they discovered an alternate use for the material—as a catalyst in more advanced types of lithium-based batteries.
Searching for fingerprints of oxygen redox
A recently developed tenet of the battery community is that battery electrodes composed of lithium-rich material can offer high-voltage, high-capacity operation because the oxygen in the material participates in reversible chemical “redox” reactions, in which oxygen atoms cyclically lose and gain electrons, helping the battery to have a higher capacity to store and use electrical charge.
At ALS Beamlines 8.0.1 and 4.0.3, resonant inelastic x-ray scattering (RIXS) can essentially dissect chemical reactions one element at a time. The researchers used this technique to map different stages of the charge-discharge cycle in lithium manganese oxide (Li2MnO3), which contains the largest amount of lithium possible within this family of materials.
The data surprisingly revealed no evidence of the “fingerprints” of the reversible oxygen redox reactions expected for this material. Instead, the researchers found that oxygen is involved in only a one-way oxidation reaction, and in very active surface reactions.
Further analysis of the other element in the material, manganese, revealed that the material can be cycled because of an unusual and complete switch to manganese-based reactions, with a relatively low capacity, right after the first charging. The finding paves the way to explorations of high-energy electrode materials outside the lithium-rich family.
A new role emerges
The research team was especially surprised to observe the “partially reversible” formation and disappearance of carbonate compounds on the surface of the material. Because the carbonate compounds contain carbon bonded to oxygen atoms, the lithium-rich material could be an effective catalyst for reactions involving CO2 gas. Such a catalyst would facilitate the reversible chemical reactions required for exotic, next-gen technologies like lithium–CO2 batteries, which would use the greenhouse gas CO2 for reversible energy conversion and storage.
Measurements of a high-capacity lithium–CO2 battery with a catalyst of Li2MnO3 showed the reactions to be highly reversible compared to similar systems with typical oxide-based catalysts. The results open the door to a whole class of alkali-rich materials to be used as catalysts for other applications, such as fuel cells.
While the study overturns several popular models for understanding oxygen redox activities in battery electrodes, it also opens up new thinking about the types of low-cost materials that can utilize oxygen redox reactions, since researchers found that the behavior of the oxygen redox reaction in lithium-rich electrodes is actually the same as that of conventional electrodes in use today, thus removing the limitation set by “lithium richness.”
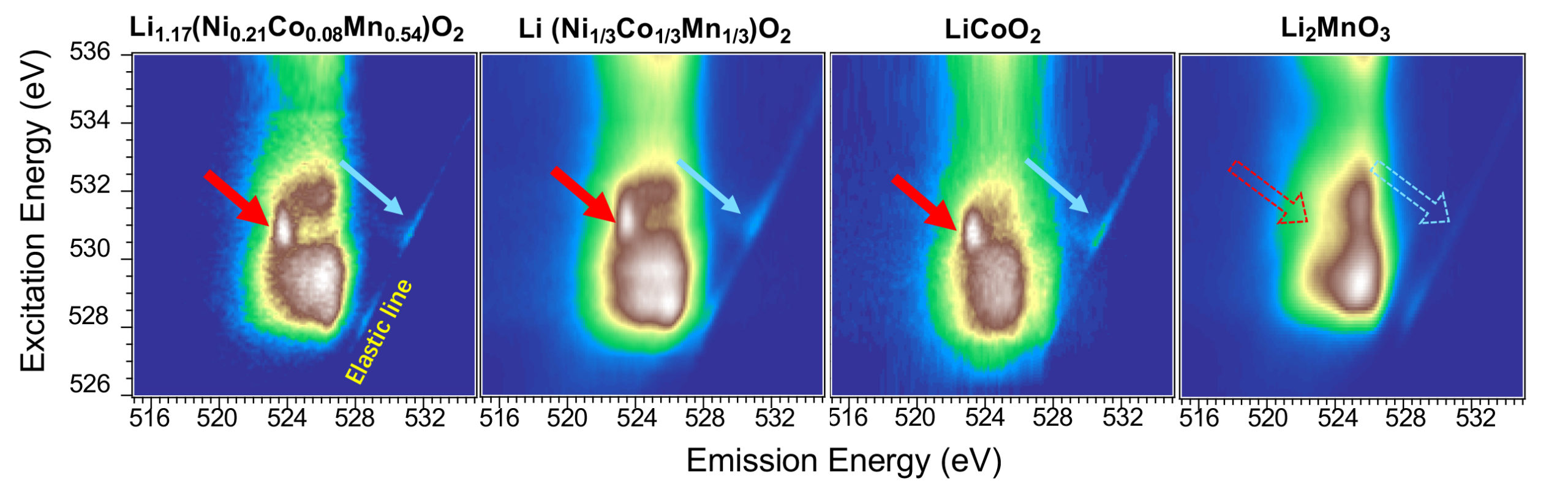
Contact: Wanli Yang
Researchers: Z. Zhuo (Peking University and ALS); K. Dai (Tianjin Normal University, China; Northeastern University, China; and ALS); R. Qiao, Y.-D. Chuang, and W. Yang (ALS); R. Wang, Y. Liu, J. Peng, L. Chen, and H. Li (Institute of Physics, Chinese Academy of Sciences); J. Wu (Stanford University, SLAC National Accelerator Laboratory, and ALS); F. Pan (Peking University); Z.-X. Shen and T.P. Devereaux (Stanford and SLAC); and G. Liu (Berkeley Lab).
Funding: National Key R&D Program of China, Beijing Municipal Science & Technology Commission, National Natural Science Foundation of China, and U.S. Department of Energy, Office of Science, Basic Energy Sciences program (DOE BES). Operation of the ALS is supported by DOE BES.
Publication: Z. Zhuo, K. Dai, R. Qiao, R. Wang, J. Wu, Y. Liu, J. Peng, L. Chen, Y.-D. Chuang, F. Pan, Z.-X. Shen, G. Liu, H. Li, T.P. Devereaux, W. Yang, “Cycling mechanism of Li2MnO3: Li–CO2 batteries and commonality on oxygen redox in cathode materials,” Joule, doi:10.1016/j.joule.2021.02.004 (2021).
Adapted from the Berkeley Lab news release, “X-Ray Study Recasts Role of Battery Material from Cathode to Catalyst.”
ALS SCIENCE HIGHLIGHT #441