SCIENTIFIC ACHIEVEMENT
With the support of data from the Advanced Light Source (ALS), researchers modified the surface of an electrocatalyst to maximize its efficiency at splitting water.
SIGNIFICANCE AND IMPACT
The optimized material is approximately 40 times more efficient than similar commercial electrocatalysts and could help make the production of clean hydrogen fuel more sustainable and economical.
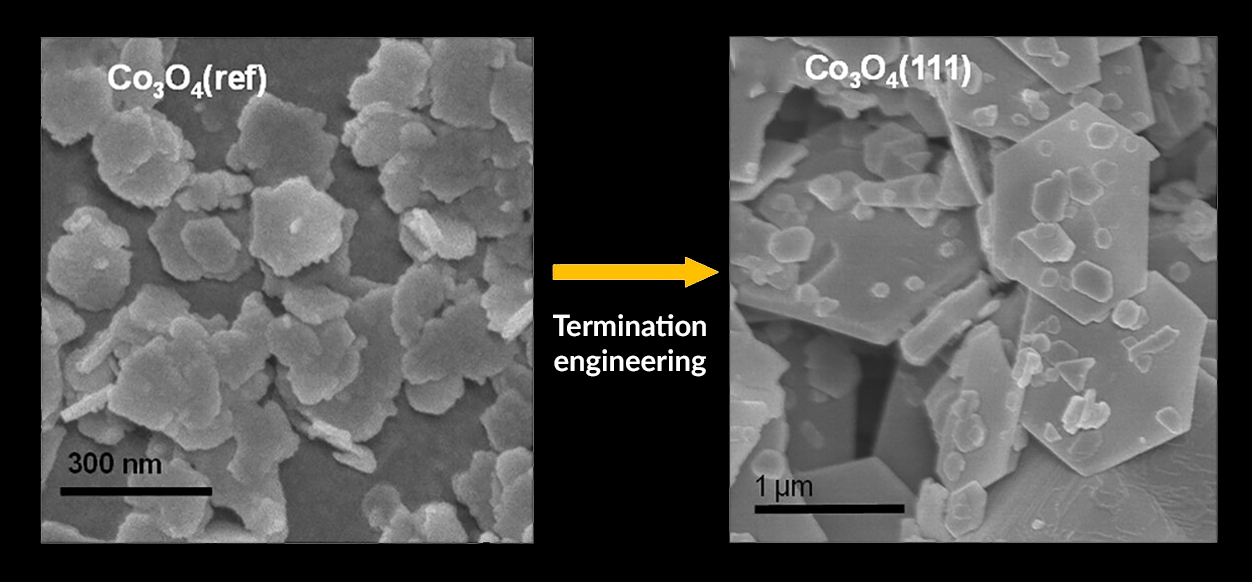
Lowering barriers to clean hydrogen
The production of hydrogen fuel through the use of renewable energy is a promising way to address the energy needs of the modern world. Currently, the process of splitting water to generate “clean” hydrogen—avoiding the input of fossil fuels and the output of greenhouse gases—requires precious-metal electrocatalysts such as platinum and ruthenium. While these materials are effective at lowering the energy barriers that slow down a key chemical reaction (the oxygen evolution reaction, or OER), they raise economic barriers because of their rarity and expense. Less costly earth-abundant materials, on the other hand, have proven less effective at driving the sluggish OER, creating a bottleneck in the generation of hydrogen.
In this work, researchers synthesized a modified form of cobalt oxide (Co3O4) that is 40 times more efficient in driving the OER than ruthenium oxide (RuO2), a commercial precious-metal electrocatalyst. Numerous experiments, including x-ray spectroscopy at the ALS, revealed key information about the material’s modified surface structure and chemical properties, advancing our understanding of oxide electrocatalysts for a wide variety of energy technologies.
Facile, faceted nanosheets
An inexpensive transition-metal oxide, Co3O4 has excellent catalytic performance and has also been used in various other energy-conversion applications such as batteries and supercapacitors. It’s known to have strong facet-dependent properties (i.e., the same crystal facet can “terminate” with different surface topographies, atomic arrangements, and electronic structures), making it an ideal platform for surface “termination engineering.” The challenge is to enable the activation of its lattice oxygen (an increasingly recognized contributor to catalytic activity) while maintaining structural stability.
Here, researchers accomplished this by synthesizing a form of Co3O4 that places a particular crystal facet—the diagonal (111) plane—at the surface. Using a facile and template-free method (molten alkaline flux), they prepared Co3O4(111) nanosheets with highly oxidized surfaces that are exclusively terminated by Co3+, which is more efficient than Co2+ in catalyzing the OER. The samples’ essential geometrical features (e.g., octahedral coordination and short Co–O bond length) were confirmed by extended x-ray absorption fine structure (EXAFS) spectroscopy and high-angle annular dark-field scanning transmission electron microscopy (HAADF-STEM).
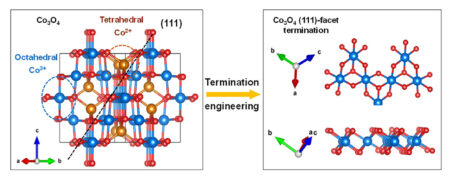
Spectroscopic studies at the ALS
At ALS Beamline 8.0.1, x-ray absorption near-edge structure (XANES) spectra of the Co L-edge and O K-edge confirmed that Co3O4(111) is exclusively terminated by octahedrally coordinated Co3+. High-efficiency mapping of resonant inelastic x-ray scattering (mRIXS) displayed broad Co–O hybridization features, together with a weak but clear “fingerprint” of a nondivalent state of the oxygen, information that can be buried in XANES spectra. Together with density functional theory calculations, the results show that the greatly improved performance of Co3O4(111) can be understood as a result of the increased surface density of highly active Co3+ and the involvement of oxygen anions as independent active sites in the OER process.
The researchers expect that a promising avenue for future investigation along these lines is the use of termination-engineered electrocatalysts in anion exchange membrane (AEM) water electrolysis cells. More broadly, the role of oxidized lattice oxygen in other lattice oxygen mechanism (LOM)-dominated materials, especially current commercially used ones, can be further investigated to find the best solution for hydrogen production.
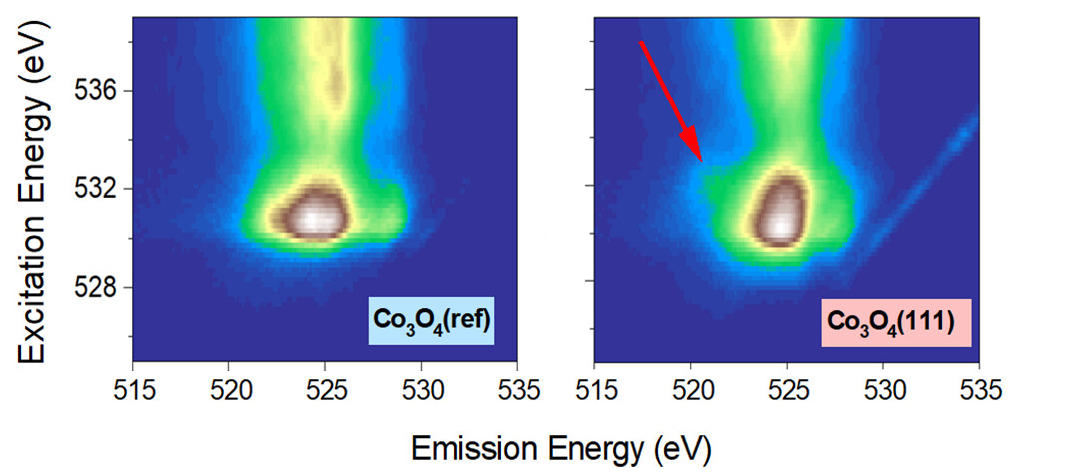
Contact: Zhenxiang Cheng
Researchers: X. Li, Y. Du, X. Wang, and Z. Cheng (University of Wollongong, Australia); L. Ge, H. Huang, Z. Fu, and Y. Lu (University of Science and Technology of China); and Y. Ha and W. Yang (ALS).
Funding: National Natural Science Foundation of China, Australia Research Council, and Anhui Provincial Department of Science and Technology (China). Operation of the ALS is supported by the US Department of Energy, Office of Science, Basic Energy Sciences program.
Publication: X. Li, L. Ge, Y. Du, H. Huang, Y. Ha, Z. Fu, Y. Lu, W. Yang, X. Wang, and Z. Cheng, “Highly Oxidized Oxide Surface toward Optimum Oxygen Evolution Reaction by Termination Engineering,” ACS Nano 17, 6811 (2023), doi:10.1021/acsnano.3c00387.
ALS SCIENCE HIGHLIGHT #486