SCIENTIFIC ACHIEVEMENT
The rate-limiting step in catalysis involving oxygen uptake was identified was identified through detailed analysis of the reaction pathways, combined with observations performed under operating conditions at the Advanced Light Source (ALS).
SIGNIFICANCE AND IMPACT
The work lays the foundation for improving the efficiency of energy conversion and storage devices such as fuel cells, catalytic reactors, and batteries.
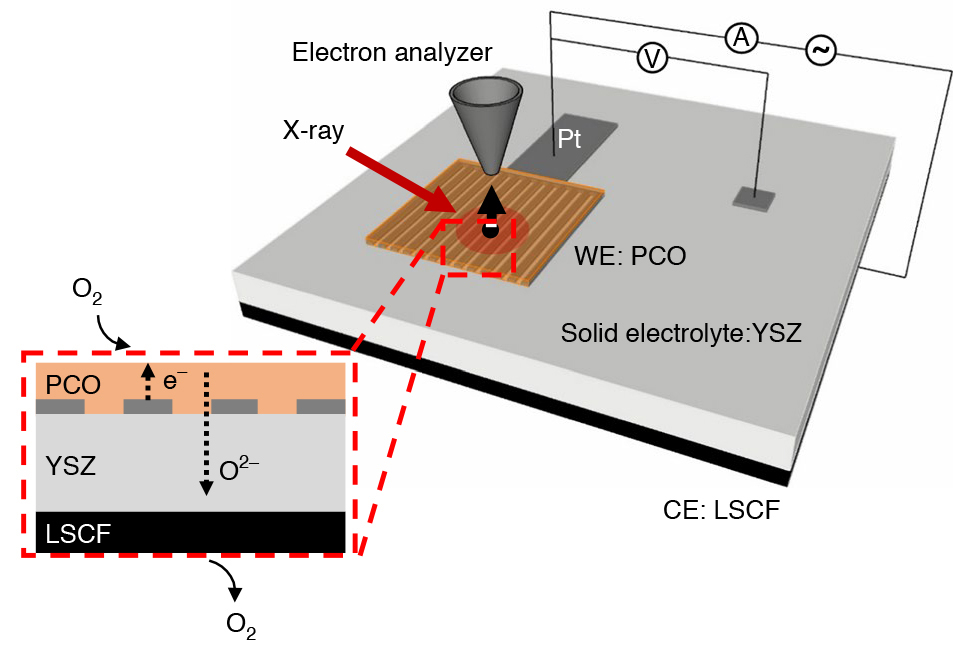
Heterogenous catalysis
Solids that catalyze chemical reactions involving gas-phase reactants and products are called heterogenous catalysts, and they are the backbone of the petrochemical industry. This type of catalyst also enables renewable-energy technologies, including fuel cells (which convert hydrogen fuel to electricity) and electrolyzers (which use electricity to generate hydrogen fuel). Thus, improvements in the efficiency of heterogeneous catalysts would help reduce the environmental impact of numerous energy-intensive processes.
Many heterogenous catalysts work well because they not only have the right geometric and electronic surface structure, but because they are also able to incorporate the gas reactant into their bulk, which enhances reaction rates. For example, many oxide catalysts, like cerium oxide, are known to take up oxygen gas (O2) in the form of oxygen ions (O2-).
Mixed ion and electron transfer
The electrochemical reduction of an oxygen gas molecule into oxygen ions requires the addition of four electrons and produces two ions, all of which cross the solid–gas interface. It’s an example of a mixed ion and electron transfer (MIET) reaction, crucial in intercalation electrochemistry, such as that occurring in lithium-ion battery electrodes.
To engineer interfaces that allow such reactions to proceed faster or more efficiently, scientists first need to identify the rate-determining step and understand it at the molecular, electronic, and point-defect levels. However, interfacial charge-transfer reactions involving both electrons and ions increase the complexity of a reaction, making it difficult to disentangle the numerous interaction pathways involved.
Operando characterization
The ambient-pressure x-ray photoelectron spectroscopy (APXPS) technique pioneered at the ALS allows heterogenous catalysts to be studied as reactions occur, under working conditions (“operando”), at temperatures and pressures not far removed from that of a real device or reactor. Scientists from Stanford University and the ALS designed an electrochemical cell to precisely tune the amount of oxygen taken up by a promising ceria-based catalyst, praseodymium cerium oxide (PCO).
As the oxygen-insertion reaction proceeded, the surface of the catalyst was probed using APXPS at ALS Beamline 9.3.2. X-ray absorption spectroscopy (XAS) was also performed under similar conditions at Beamline 11.0.2 to determine the near-surface oxidation state of the PCO as a function of external voltage. Finally, the reaction rate was determined precisely by measuring the electrical current that passed through the electrochemical cell.
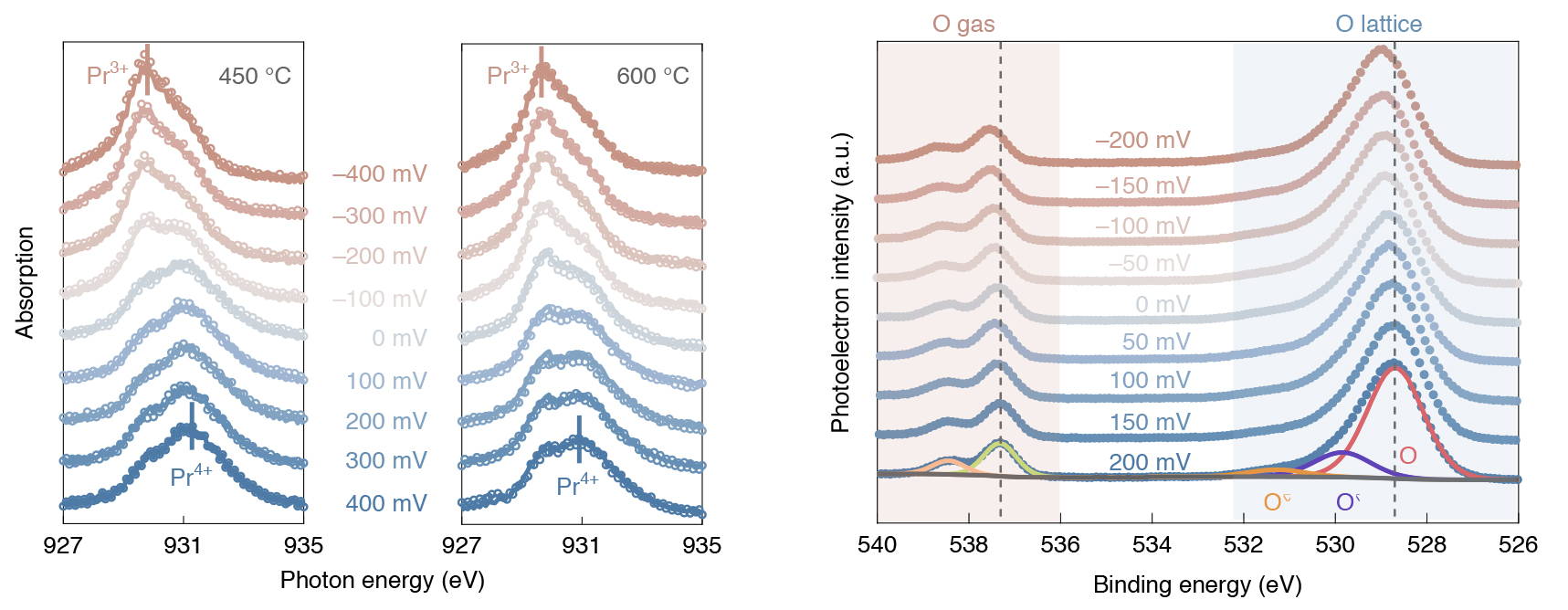
Converging on the bottleneck
The experimental data, which quantified the system’s chemical and electrostatic driving forces, was combined with a microkinetic model that describes the relationship of the reaction rate to the amount of oxygen incorporated into the catalyst. This powerful combination enabled the researchers to precisely pinpoint the step, out of more than 100 possibilities involving the transfer of four electrons and two ions, that was limiting the overall reaction rate: the dissociation of the neutral molecular oxygen on the surface.
This important insight will now be used to further improve catalysts for oxygen reduction in solid-oxide fuel cells (for directly converting hydrogen and natural gas to electricity). More generally, the combination of microkinetic model and operando characterization via APXPS is emerging as a powerful platform for studying a wide range of catalytic reactions and for zeroing in on the bottlenecks in complex catalytic reactions.
Contact: Will Chueh
Researchers: D. Chen, Z. Guan, D. Zhang, and W.C. Chueh (Stanford University); L. Trotochaud, S. Nemsak, and H. Bluhm (Berkeley Lab); E. Crumlin (ALS); and H.L. Tuller (Massachusetts Institute of Technology).
Funding: National Science Foundation and U.S. Department of Energy, Office of Science, Basic Energy Sciences program (DOE BES). Operation of the ALS is supported by DOE BES.
Publication: D. Chen, Z. Guan, D. Zhang, L. Trotochaud, E. Crumlin, S. Nemsak, H. Bluhm, H.L. Tuller, and W.C. Chueh, “Constructing a pathway for mixed ion and electron transfer reactions for O2 incorporation in Pr0.1Ce0.9O2−x,” Nat. Catal. 3, 116 (2020), doi:10.1038/s41929-019-0401-9.
ALS SCIENCE HIGHLIGHT #419