Protein phosphorylation regulates protein function in a cell, either activating or inactivating the proteins responsible for many cell functions ranging from cell proliferation to differentiation to metabolism to signaling, and even programmed cell death. This chemical process has been studied intensively, but until now it has been impossible to watch phosphorylation at the molecular level without damaging cells or interfering with the very processes being examined. Using ALS Infrared Beamline 1.4, a group of researchers led by Hoi-Ying Holman, director of the Berkeley Synchrotron Infrared Structural Biology program at the ALS, developed a new technique for monitoring protein phosphorylation inside living mammalian cells, enabling them to follow cellular chemical changes in real time, without bias.
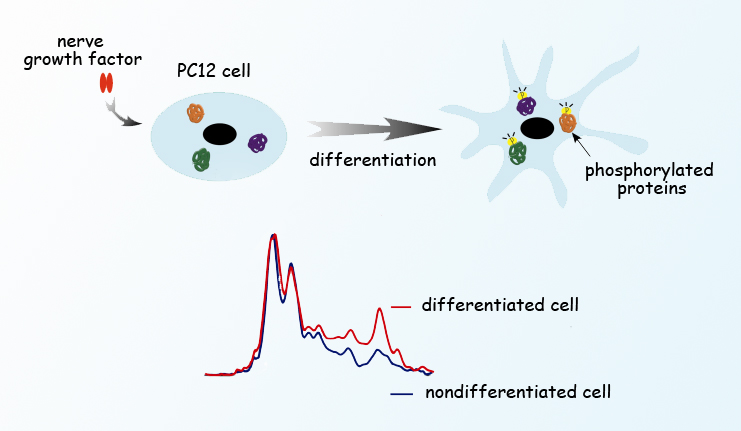
Phosphorylating enzymes add one or more phosphate groups to three amino-acid residues common in proteins—serine, threonine, or tyrosine—which activates the proteins; removing the phosphate reverses the process. Previous research into phosphorylation was done by labeling specific phosphorylated proteins with antibodies that carry fluorescent dyes, requiring that the researcher know exactly what to label before beginning. Now, Holman and colleagues from the University of California, Berkeley, and San Diego, have figured out how to track cellular chemical changes without preconceived notions of what they might be.
To avoid killing cells or introducing modified proteins or foreign bodies that may alter their behavior, scientists used a method called Fourier-transform infrared (FTIR) spectromicroscopy. Because infrared light has lower photon energy than x-rays, it can be used to peer inside living cells without damaging them. Different components of the cell absorb different wavelengths of the broad infrared spectrum; applying the Fourier-transform algorithm allows signals of all frequencies to be recorded simultaneously, pinpointing when, where, and what chemical changes are occurring. Infrared from the ALS’s synchrotron light source is a hundred to a thousand times brighter than typical infrared sources. To learn exactly when proteins and receptors are switched on and off by phosphorylation, and which cells within a population are responding with changes, scientists monitored unlabeled living cells by studying the absorption of a wide spectrum of synchrotron infrared radiation from the ALS, tracking the cells over a week’s time as they underwent a series of major changes.
The study was done using a line of cultured cells called PC12. When nerve growth factor, a small protein, is introduced into a PC12 cell, the cell begins to send out neurites resembling the projections from nerve cell bodies. PC12 has become a valuable model of how nerve cells differentiate from their unspecialized progenitors. The cells were cultured on gold-coated slides in a humidified environment, in chambers maintained at body temperature and supplied with nutrients. Scientists induced differentiation by introducing nerve growth factor to groups of PC12 cells. Individual cells of a group were positioned under the infrared beam, and FTIR spectra were collected before and after the nerve growth factor was introduced. After stimulation, the spectra were taken first at short intervals, from two to sixty minutes apart. Additional spectra were collected from cells in other groups on the third, fifth, and seventh day of continued stimulation.
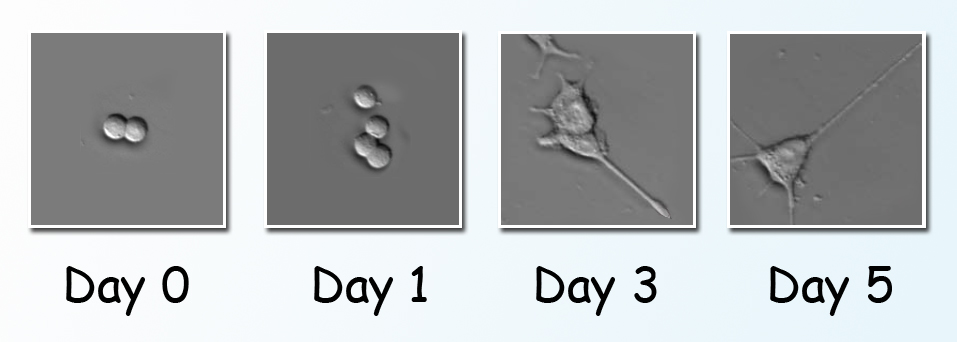
The first day’s spectra revealed spikes in phosphorylation activity only minutes after nerve growth factor was added, coinciding with changes in the ratios of important cellular materials like proteins, carbohydrates, and lipids. Phosphorylation subsequently waned, then picked up again in another burst of activity on day 3, just as the cells began to extend neurites. By comparing results with quantum chemistry simulations—predicting what should be observed from first principles—as well as with results from partial studies using other methods, the researchers confirmed the monitoring of phosphorylation phases, their timing, and their target proteins, along with associated changes in other substances in the cell.
Although the team was not able to follow individual cells continuously, they were able to monitor differentiation in groups of cultured PC12 cells in real time, without labeling or any other invasive procedure. It was the first step in an ambitious inquiry into the real-time biochemistry of living mammalian cells over the long term, and the coordination of specific processes in real time.
At ALS Beamline 1.4 the team is designing equipment to maintain mammalian cells in a thin layer of culture media that will keep them healthy but will not interfere with the infrared beam, while automatically monitoring and adjusting temperature, humidity, and nutrient ratios, and removing waste products. This will allow data on individual cells to be gathered continuously throughout the entire phosphorylation process.
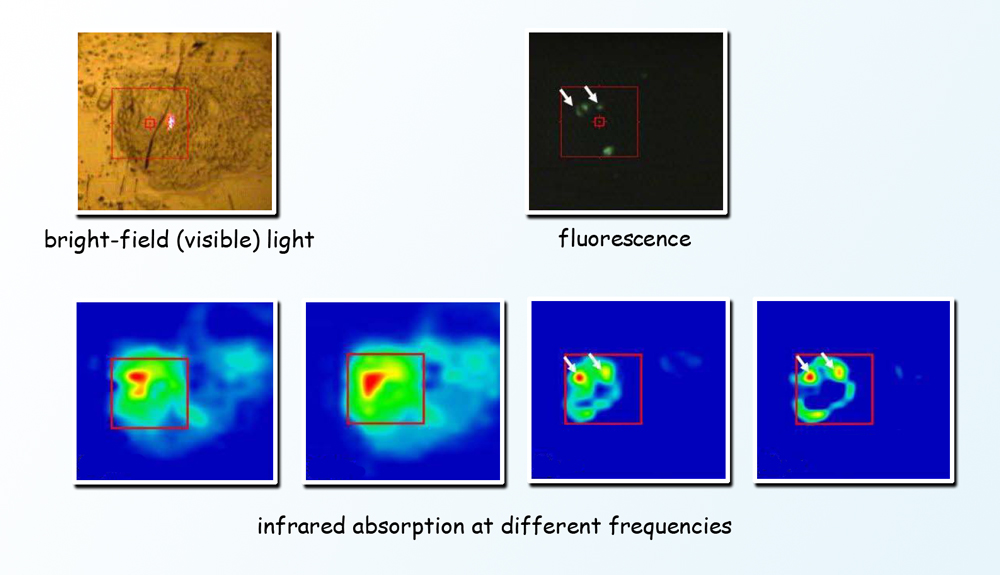
Contact: Hoi-Ying Holman
Research conducted by: L. Chen, H.-Y.N. Holman, Z. Hao, H.A. Bechtel, and M.C. Martin (Berkeley Lab), C. Wu (University of California, San Diego), and S. Chu (University of California, Berkeley).
Research funding: Operation of the ALS and the Berkeley Synchrotron Infrared Structural Biology program are supported by the U.S. Department of Energy, Office of Basic Energy Sciences.
Publication about this research: L. Chen, H.-Y.N. Holman, Z. Hao, H.A. Bechtel, M.C. Martin, C. Wu, and S. Chu, “Synchrotron infrared measurements of protein phosphorylation in living single PC12 cells during neuronal differentiation,” Analytical Chemistry 84, 9 (2012).
ALS SCIENCE HIGHLIGHT #257