SCIENTIFIC ACHIEVEMENT
Using a multimodal approach developed at the Advanced Light Source (ALS), researchers learned how chemical properties correlate with structural changes during nanoparticle growth.
SIGNIFICANCE AND IMPACT
The work will enable a greater understanding of the mechanisms affecting the durability of nanoparticles used to catalyze a broad range of chemical reactions, including clean-energy reactions.
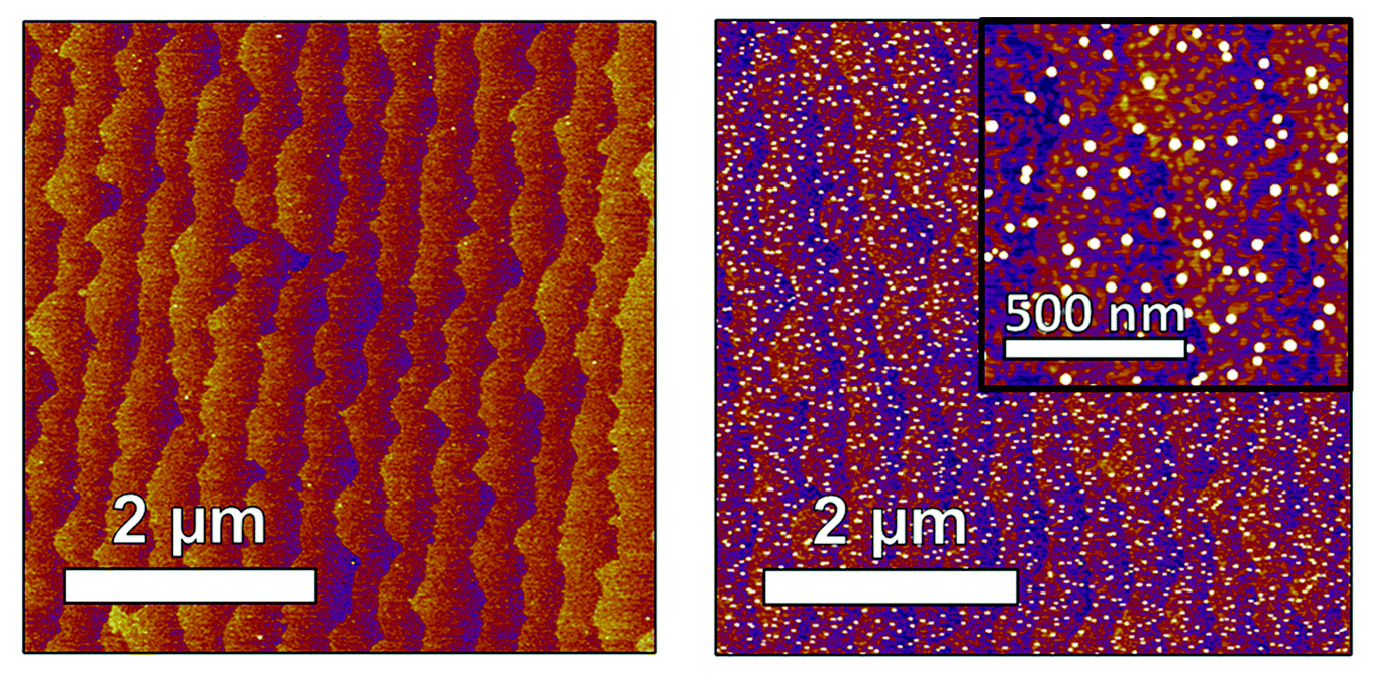
Catalyzing technological progress
In applications ranging from chemical synthesis to energy storage, catalysts enable chemical reactions to run at more favorable temperatures, pressures, or in general, with lower energy requirements. For example, catalysts enable the efficient splitting of water to generate hydrogen, which can then be used as a clean, decarbonized fuel.
For such applications, nanoparticles on the surface of a transition-metal oxide work well as catalysts, but they are susceptible to coarsening, agglomeration, and other forms of degradation, shortening their usable lifetime. In this work, researchers applied a technique they developed at the ALS to simultaneously study the chemistry and structure of catalyst materials as they form, a capability that will help scientists identify strategies for improving nanoparticle durability.
Understanding nanoparticle exsolution
A process called “exsolution” has shown significant promise for controlling nanoparticle size, shape, distribution, and stability. Briefly, the process involves causing dopant atoms in a host matrix to migrate to the surface and gather to form nanoparticles. This is done by heating the host material under reducing conditions (i.e., in a reducing gas such as hydrogen). Exsolution from metal oxide hosts produces highly stable metal nanoparticles that are often partially embedded in the oxide surface and show high activity for the oxygen evolution reaction (OER), a key step in many electrochemical reactions, including water splitting.
Here, the samples studied were thin films of SrTi0.9Nb0.05Ni0.05O3-δ (STNNi). When STNNi is heated in H2 gas, the Ni atoms migrate to the surface and form nanoparticles. Before the reducing treatment, such samples are inactive with respect to the OER. After treatment, the system becomes active, despite a relatively small amount of Ni doping.
Correlated photoemission and scattering
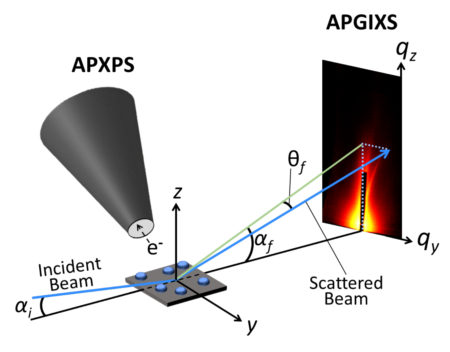
To better understand the chemical and structural transformations occurring in the Ni nanoparticles during exsolution, the researchers applied a technique they developed at ALS Beamline 11.0.2, dubbed Ambient-Pressure PhotoEmission and X-ray Scattering (APPEXS). This new capability, currently available only at the ALS but being developed at other facilities around the world, allows simultaneous ambient-pressure x-ray photoelectron spectroscopy (APXPS) and ambient-pressure grazing-incidence x-ray scattering (APGIXS) measurements, enabling the correlation of chemical and structural changes, respectively, as the nanoparticles grow.
The researchers also used scanning probe microscopy to establish that nanoparticle exsolution occurred and obtain boundary information about particle morphologies and distributions for use in computer modeling. The elemental composition of the Ni nanoparticles was supported by nanoprobe x-ray absorption spectroscopy at the Advanced Photon Source.
The exsolution solution
The correlated data showed that, as nanoparticle size and structure evolved, the surface Ni component transformed from oxide to metal, while the bulk of the STNNi film was not affected. Particle morphology simulations based on the scattering data revealed that the particles grew larger and adopted a compressed shape as exsolution proceeded.
The work serves as an important proof of principle for studies of exsolved materials as they’re grown or during reactions, and it opens up new avenues for studying complex systems in realistic environments. The researchers are now exploring collaborations to apply this technique to diverse scientific areas, including atmospheric science, microelectronics, corrosion science, hydrogen storage, and other heterogeneous catalytic systems.
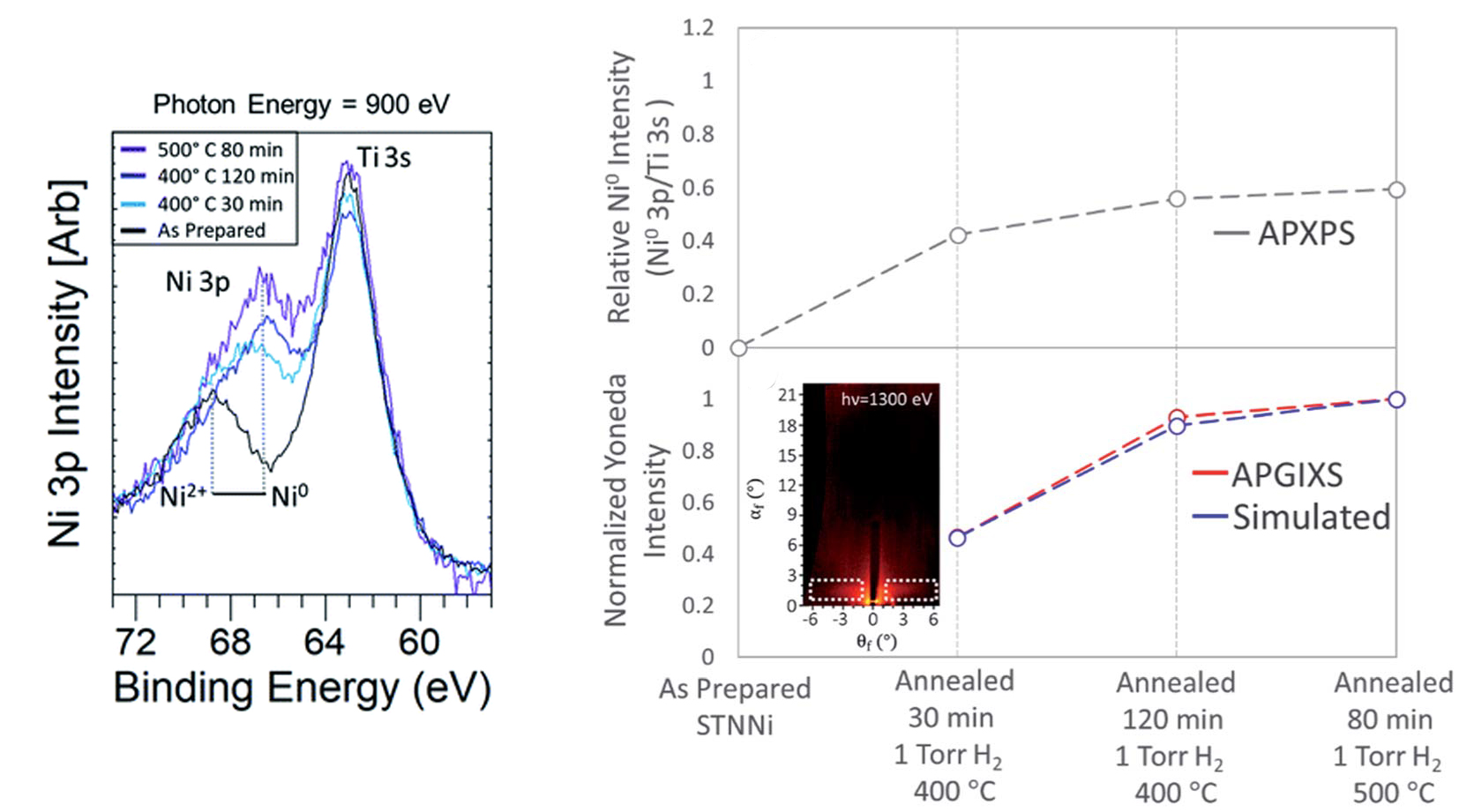
Contacts: Slavo Nemsak, Heath Kersell, and Moritz Weber
Researchers: H. Kersell, Q. Lu, and S. Nemšák (ALS); M.L. Weber, C. Lenser, and F. Gunkel (Forschungszentrum Jülich, Germany); L. Falling (Berkeley Lab); C. Baeumer (University of Twente, The Netherlands); and N. Shirato and V. Rose (Argonne National Laboratory).
Funding: Laboratory Directed Research and Development Program (Berkeley Lab) and Alexander von Humboldt Foundation. Operation of the ALS and APS is supported by the U.S. Department of Energy, Office of Science, Basic Energy Sciences program.
Publication: H. Kersell, M.L. Weber, L. Falling, Q. Lu, C. Baeumer, N. Shirato, V. Rose, C. Lenser, F. Gunkel, and S. Nemšák, “Evolution of surface and sub-surface morphology and chemical state of exsolved Ni nanoparticles,” Faraday Discuss. 236, 141 (2022), doi:10.1039/D1FD00123J.
ALS SCIENCE HIGHLIGHT #473