SCIENTIFIC ACHIEVEMENT
With the help of the Advanced Light Source (ALS), researchers learned how molecular structure relates to function in catalysts that convert atmospheric nitrogen into more usable forms at room temperature and pressure.
SIGNIFICANCE AND IMPACT
The work could lead to greater energy efficiency in producing nitrogen-based products such as fertilizer where large-scale industrial processes are unfeasible.
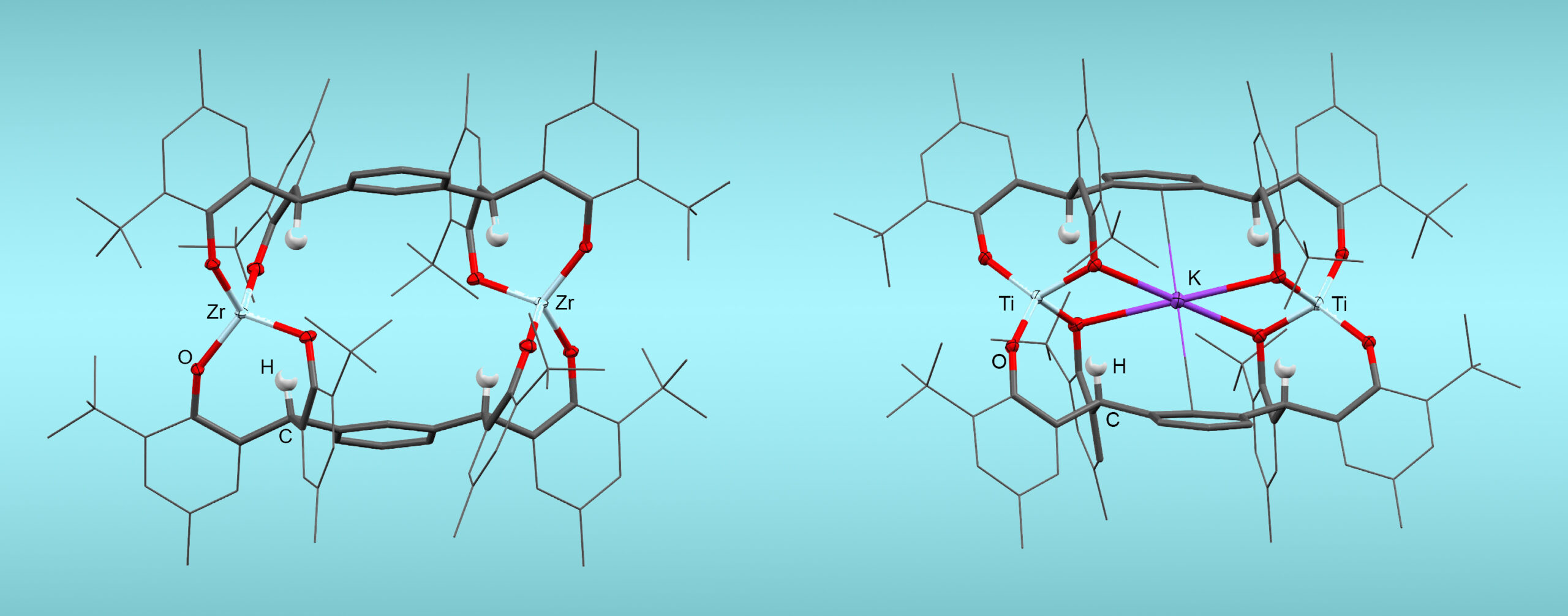
Coaxing nitrogen from the air
Ammonia is the starting point for the fertilizers that have secured the world’s food supply for the last century. But synthesizing ammonia (NH3) from atmospheric nitrogen (i.e., dinitrogen, N2) requires a high-temperature, high-pressure industrial process known as the Haber-Bosch process. It accounts for around 2% of global energy use and creates geographic inequities in the availability of ammonia where energy supplies are limited.
Now, researchers led by Polly Arnold, a senior staff scientist and director of Berkeley Lab’s Chemical Sciences Division, have found catalysts that can facilitate N2 reduction (i.e., conversion to more usable forms) at room temperature and pressure. Although this technology won’t replace the Haber-Bosch process at a large scale, it could improve food and energy security by bringing fertilizers and other nitrogen products to remote regions at a much lower cost.
A cavity for catalytic activity
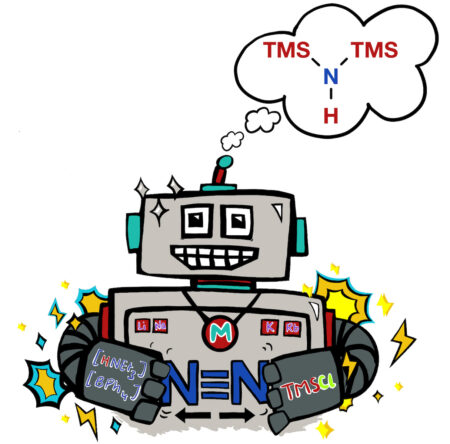
The researchers designed molecular complexes that feature a rectangular cavity within a framework of organic ligands. The cavity serves as the active site for N2 reduction, with the reaction being mediated by cheap, earth-abundant metal cations—either group 4 elements (titanium or zirconium) or lanthanides (samarium or cerium)—located on opposite sides of the cavity.
Dinitrogen that diffuses into the cavity forms bonds with the metals, which activates the gas. Electrons introduced into the cavity by potassium (an electron donor, or “reductant”) attack the activated nitrogen, cleaving its bonds. In all its standard forms, reduced nitrogen makes three covalent bonds to hydrogen atoms or other reactants such as trimethyl silyl (TMS)—a functional group with a central silicon bonded to three methyl (CH3) groups. These ammonia derivatives can then be used to synthesize other desired products.
Characterization and analysis
To gain a better understanding of how the metal cations, reductant, and ligand architecture work cooperatively to control the selective reduction of N2, the complexes were crystallized and their structures were determined, using both lab- and synchrotron-based single-crystal x-ray diffraction. The latter, performed at ALS Beamline 12.2.1, provided key information about cavity dimensions in intermediate compounds that form in the presence of the potassium reductant. Other experimental techniques used in the analysis included cyclic voltammetry, mass spectroscopy, gas chromatography, several different types of spectroscopy, and computational modeling.
How form influences function
The results showed that the group 4 complexes (with titanium or zirconium) featured smaller cavities and selectively produced bis(silyl)amine (i.e., nitrogen bonded to one hydrogen and two TMS groups), while the lanthanide complexes (with samarium or cerium) had larger cavities that could make both bis(silyl)amine and tris(silyl)amine (i.e., nitrogen bonded to three TMS groups)—all under ambient conditions.
The researchers next intend to use electrodes as a source of electrons, which could enable nitrogen reduction using renewable solar energy. They also plan to use x-ray absorption spectroscopy (XAS) and resonant inelastic x-ray scattering (RIXS) at the ALS to understand which lanthanide properties affect the reaction chemistry, with the goal of synthesizing additional nitrogen-containing products by tuning the shape and size of the cavity.
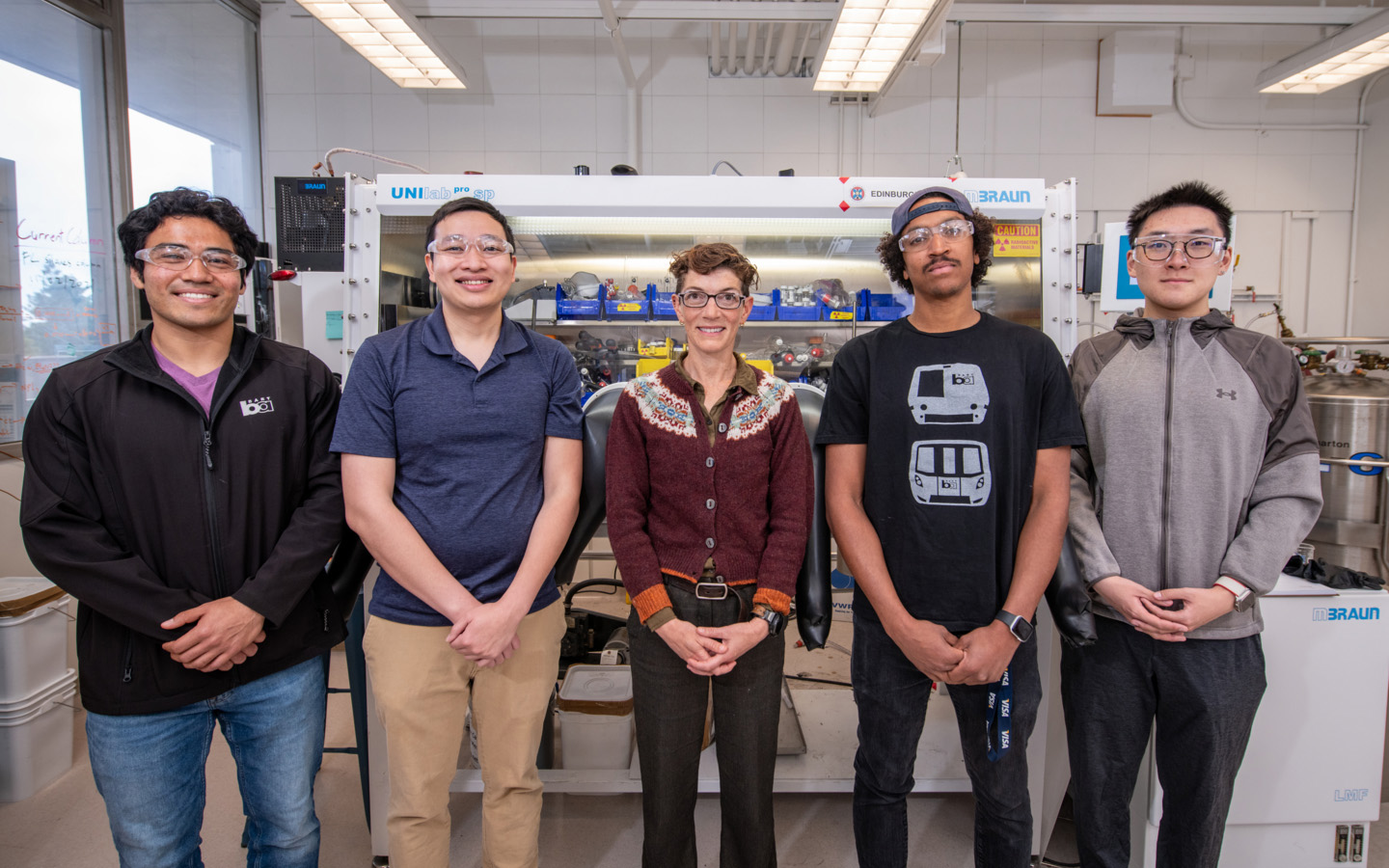
Contact: Polly Arnold
Researchers: A. Wong, F.Y.T. Lam, M. Hernandez, J. Lara, T.M. Trinh, R.P. Kelly, T. Ochiai, and P.L. Arnold (Univ. of California, Berkeley, and Berkeley Lab); G. Rao and R.D. Britt (Univ. of California, Davis); and N. Kaltsoyannis (University of Manchester, UK).
Funding: National Science Foundation; US Department of Energy (DOE), Office of Science, Basic Energy Sciences (BES) program; National Institutes of Health; Berkeley Lab; University of Manchester; Engineering and Physical Sciences Research Council (EPSRC), UK; and the European Research Council. Operation of the ALS is supported by DOE BES.
Publication: A. Wong, F.Y.T. Lam, M. Hernandez, J. Lara, T.M. Trinh, R.P. Kelly, T. Ochiai, G. Rao, R.D. Britt, N. Kaltsoyannis, and P.L. Arnold, “Catalytic reduction of dinitrogen to silylamines by earth-abundant lanthanide and group 4 complexes,” Chem Catal. 4, 100964 (2024), doi:10.1016/j.checat.2024.100964.
Adapted from the Berkeley Lab news release, “A Cleaner Way to Produce Ammonia.”
ALS SCIENCE HIGHLIGHT #508