SCIENTIFIC ACHIEVEMENT
Using multiple x-ray characterization tools at the Advanced Light Source (ALS), researchers showed how chemical and structural changes improve the performance of a novel ion-conducting polymer (ionomer) membrane from 3M Company.
SIGNIFICANCE AND IMPACT
The work provides insight into the factors impacting the proton conductivity of ionomers used for fuel cells and the production of hydrogen fuel.
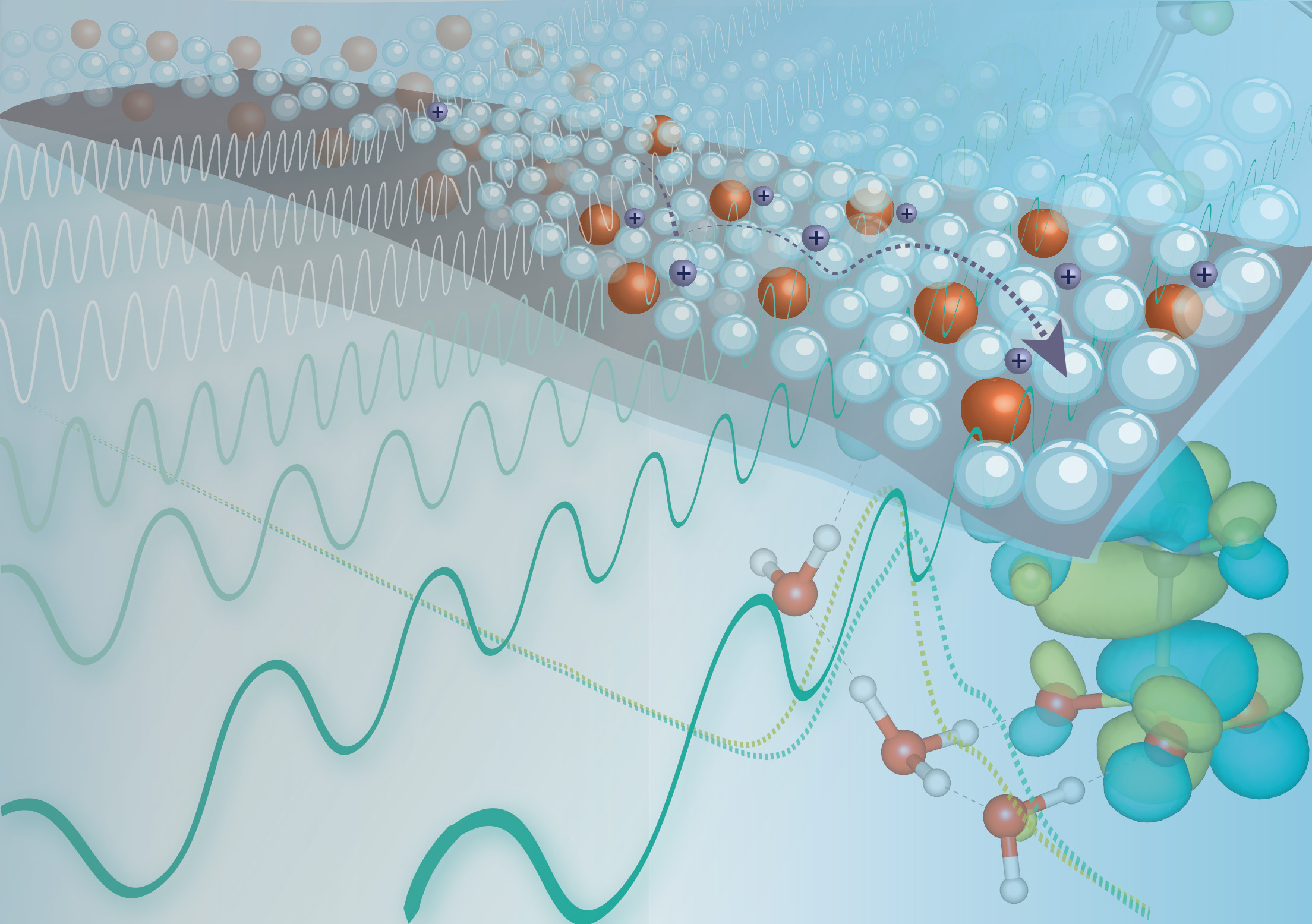
Membranes in the middle
In fuel cells (which generate clean power from hydrogen fuel) and electrolyzers (water-splitting devices that produce hydrogen fuel), positive and negative electrodes are separated by membranes composed of ion-conducting polymers (ionomers). These membranes prevent contact between the electrodes—thus avoiding catastrophic failure—while allowing selective passage of ions to complete the circuit.
Generally, such membranes are based on a class of perfluorosulfonic acid (PFSA) ionomers with remarkable proton conductivity and stability. Recently, however, companies such as 3M have been developing new ionomers with improved performance. In this work, researchers took a closer look at the structural and chemical properties of these materials at the nanometer scale. The resulting insights provide valuable guidance on design strategies for optimally performing ionomers.
Altered side-chain chemistry
The advantageous properties of PFSA membranes are attributed to a hydration-driven, phase-separated nanoscale domain structure. In the presence of water, the hydrophilic domains enclosed by the hydrophobic polymer backbone swell. The backbone imparts stability while the side chains, terminated by sulfonate (SO3–) “active sites” capable of hosting protons (H+), facilitate proton transfer across the membrane.
Because device efficiency can be improved through higher-temperature operation, researchers are interested in membranes that work in hotter, drier environments. One of 3M’s new class of ionomers, perfluoro ionene chain extended (PFICE), has multiple active sites per side chain compared to PFSA’s one, and performs well at both higher and lower relative humidity. To better understand why, a collaboration between researchers from 3M, Berkeley Lab’s Energy Storage and Distributed Resources Division, and the ALS took a multimodal approach to exploring the interplay between chemistry, structure, and function in these new materials.
Tender x-ray spectroscopy and scattering
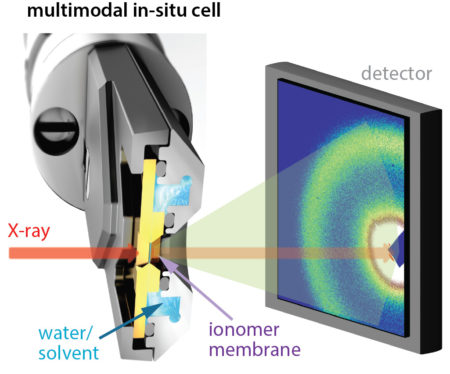
At ALS Beamline 5.3.1 (dedicated to research and development), the team used tender x-rays to perform x-ray absorption spectroscopy (XAS) and resonant x-ray scattering (RXS) at the sulfur K-edge. Tender x-rays can penetrate the relatively thick membranes of industrial interest (20 to 50 microns), and XAS techniqes reveal distinct functional groups and hydration levels at the active sites. RXS allowed the researchers to zero in on characteristic length scales (correlation lengths) of the active sites, a measure of domain connectivity. The ALS’s deep expertise in resonant scattering was thus crucial to the success of this work.
The researchers studied PFICE and PFSA membranes in air and water. A transferable flow cell enabled measurements of the same samples at multiple beamlines. Small-angle x-ray scattering (SAXS) at Beamline 7.3.3 and resonant soft x-ray scattering (RSoXS) at Beamline 11.0.1.2 corroborated the findings. First-principles calculations, using methods developed at the Molecular Foundry and performed at NERSC, helped distinguish between distinct sulfur atoms in PFICE side chains.
Proton highways with improved connectivity
The results showed that PFICE had overall greater domain connectivity than PFSA in both dry and hydrated states: the chemical changes produced a well-connected proton-transport network with more direct pathways. Moreover, the active sites were more effective at releasing protons. And, under drier conditions, the PFICE held onto more water molecules in a more evenly distributed way.
In the immediate future, the researchers plan to perform operando electrochemical experiments using the flow cell, while also varying the relative humidity and temperature. Longer term, the enhanced coherence provided by the ALS Upgrade (ALS-U) and a new tender x-ray beamline will enable scattering studies capable of capturing the dynamics of ions and polymers and will play a significant role in improving our understanding of these materials.
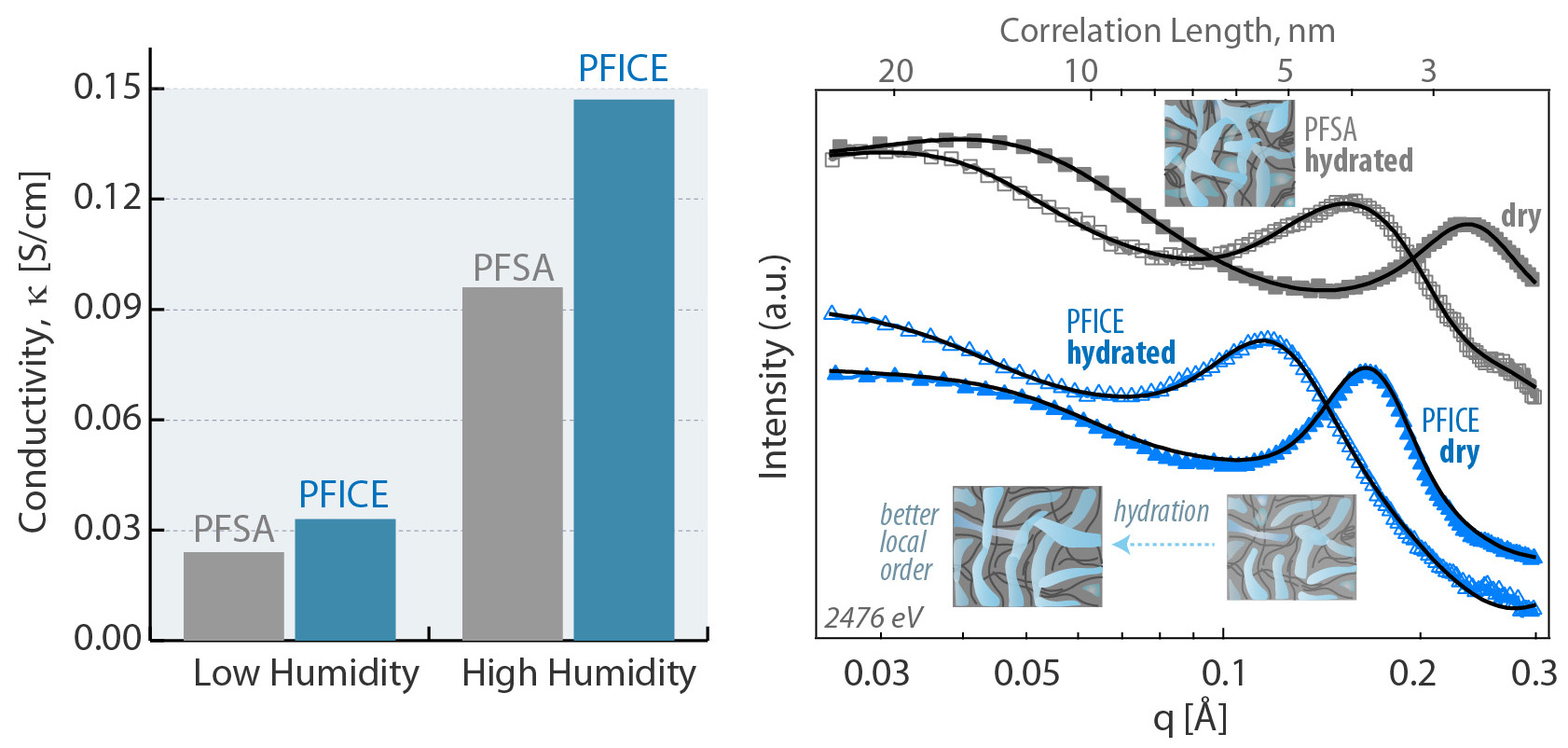
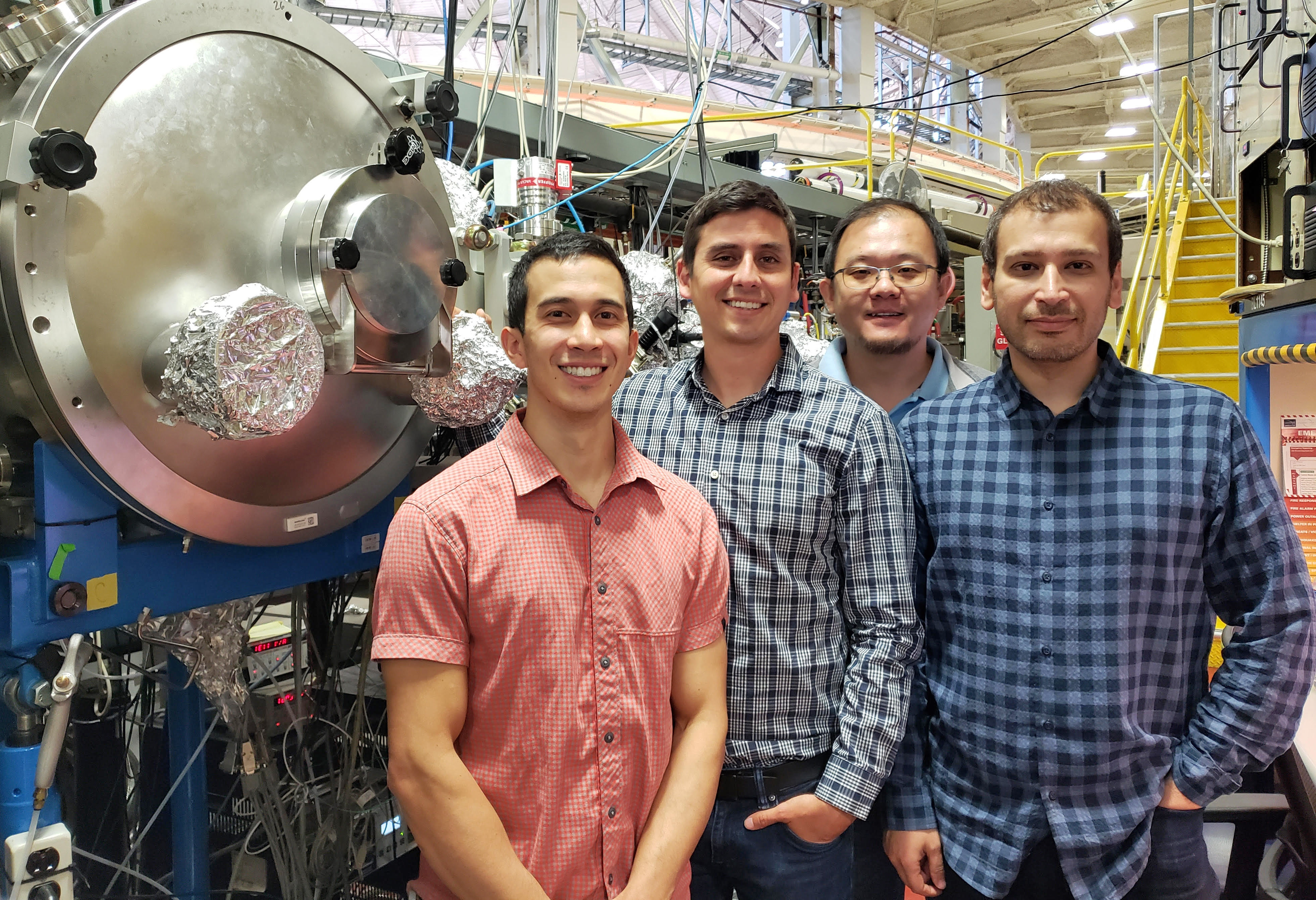
Contacts: Ahmet Kusoglu and Cheng Wang
Researchers: G.M. Su, I.A. Cordova, J. Feng, and C. Wang (ALS); M.A. Yandrasits and M. Lindell (3M Corporation); and A. Kusoglu (Berkeley Lab).
Funding: U.S. Department of Energy (DOE), Office of Energy Efficiency and Renewable Energy, Fuel Cell Technologies Office, Fuel Cell Performance and Durability (FC-PAD) consortium. Operation of the ALS is supported by DOE Office of Science, Basic Energy Sciences Program.
Publication: G.M. Su, I.A. Cordova, M.A. Yandrasits, M. Lindell, J. Feng, C. Wang, and A. Kusoglu, “Chemical and Morphological Origins of Improved Ion Conductivity in Perfluoro Ionene Chain Extended Ionomers,” J. Am. Chem. Soc. 141, 13547 (2019), doi:10.1021/jacs.9b05322.
ALS SCIENCE HIGHLIGHT #407