The burning of fossil fuels and the consequent rising levels of atmospheric CO2 has led to a number of negative environmental consequences, including global warming and ocean acidification. Converting CO2 to fuels or chemical feedstock, ideally through the use of renewable energy, can simultaneously reduce atmospheric CO2 and decrease fossil-fuel consumption. The principal difficulty in this process is that earth-abundant catalysts that can perform this reaction with high efficiency and selectivity are not readily available. Recently, however, researchers have made headway in this direction by utilizing superporous molecular structures—metal-organic frameworks (MOFs) and covalent-organic frameworks (COFs)—as a new class of CO2 reduction catalysts. This work, which incorporates structural and electronic studies performed at the ALS, gives rise to an entirely new basis in which to develop electrocatalysts for converting captured carbon dioxide into valuable chemical products such as fuels, pharmaceuticals, and plastics.
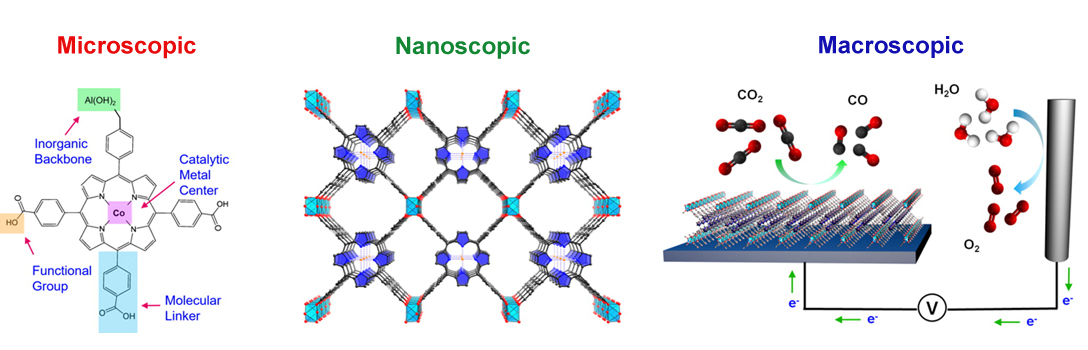
Finding electrocatalysts that can convert captured CO2 into fuels such carbon monoxide, methanol, or methane with high efficiency and selectivity has been a longstanding challenge in chemistry and engineering. The principal difficulty is that the CO2 reduction reaction is kinetically sluggish, as it is competing with water reduction. Through the reduction reaction it is possible to generate a wide variety of products and difficult to find a catalyst that’s selective for a specific one. Within this context, homogeneous molecular catalysts can offer high selectivity through precise molecular-level tuning, but typically suffer from issues of stability and solubility. On the other hand, heterogeneous catalysts like metals are more stable, but cannot be modified easily on a molecular level and therefore don’t offer product selectivity or routes for systematic improvement.
Against this backdrop, researchers have used ALS capabilities to develop a new class of CO2 reduction catalysts in the form of an organic framework that takes the advantage of both worlds. By linking organic catalytic units in an ordered, three-dimensional structure, MOFs and COFs effectively heterogenize a tunable molecular catalyst and stabilize it within the framework structure. These organic catalysts are not typically stable or soluble, but within the context of a MOF or COF these catalysts are stabilized in a solid skeleton but still available for catalysis. MOF- and COF-based catalysts also exhibit a further set of modularity in framework composition and porosity. They can be modified to exquisitely balance the concentration of active sites with mass and charge transport for a truly mesoscopic material design. Mesoscopic design allows researchers to tailor their structural designs across multiple length scales—the limitations discovered in one generation of the catalyst can potentially be solved within the next generation. The structure of MOF- and COF-based catalysts allows tailoring within the atomic level (the active site itself), the nanoscopic level (the framework and porosity), and the mesoscopic level (the overall device functioning).
MOF catalysts were synthesized by converting nanometer-thickness films of metal oxides that were deposited onto substrates by atomic layer deposition (ALD). This ALD-to-MOF method allows for precise variation of MOF thickness by controlling that of the starting material. Furthermore, many MOF structures can be fabricated by varying the oxide film composition of the molecular linker. Grazing incidence wide angle x-ray scattering (GIWAXS) measurements at ALS Beamline 7.3.3. showed that the MOF films were crystalline and preferentially oriented, with cobalt porphyrin units sitting perpendicular to the substrate. MOFs can be created using almost any organic linker, but in this case the porphyrin acted as both the catalyst and the linker. The final MOF catalyst consisted of cobalt metallated porphyrins in an aluminum oxide inorganic skeleton and converted CO2 to with a rate of 76 percent carbon monoxide as the end product. This result is among the highest ever reported in aqueous electrolytes.
COF catalysts feature a conductive framework that allows charges to easily move throughout the structure. Interestingly, the cobalt porphyrin active sites that were embedded within the COF framework were 26 times more active than the molecular cobalt porphyrin analogue. This was very surprising and unusual, as it was almost like a cooperative effect in that the catalyst and active site were the same. Within the COF, the electronic structure of the catalyst is actually modified by the framework. X-ray absorption measurements at ALS Beamline 10.3.2. provided evidence that the electronic structure of the cobalt in the COF framework may be different than that of the cobalt in the molecular linker, which is why the porphyrin was 26 times more active. With a small catalyst loading to push the limits of intrinsic catalytic activity, the cobalt porphyrin-functionalized COF attained a per-site turnover rate of 300,000.
The development of this new class of porous electrocatalysts is especially promising because the nature of MOFs and COFs allows for precise control of the active site, degree of porosity, and framework composition . Further research efforts, such as establishment of structure-function relationships and the exploration of additional frameworks, active sites, and functional groups are underway. This research could lead to a viable way to transform captured CO2 on a lab and industrial scale.
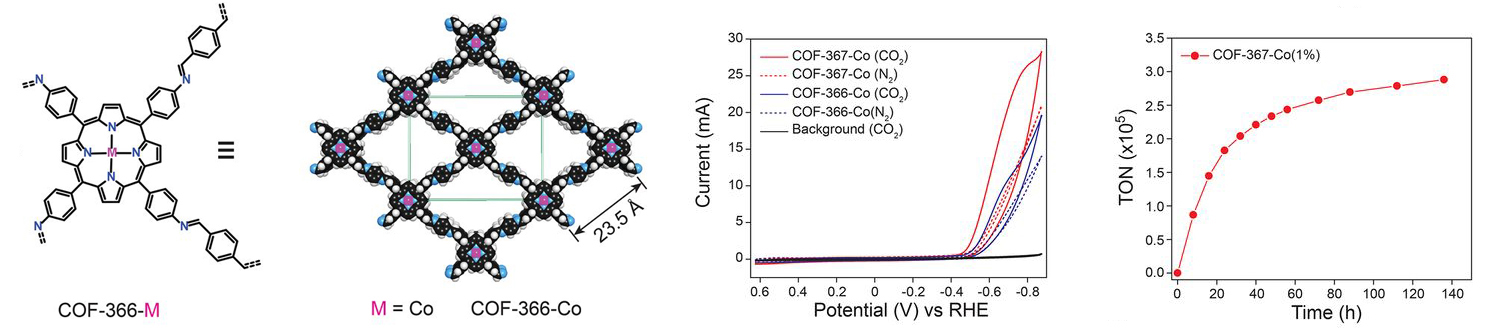
Contact: Nick Kornienko
Research conducted by: S. Lin, C.S. Diercks, E.M. Nichols (UC Berkeley, Berkeley Lab); Y.B. Zhang (UC Berkeley, Berkeley Lab, ShanghaiTech University); N. Kornienko, Y. Zhao, A.R. Paris, D. Kim, C.S. Kley (UC Berkeley); C. Zhu (Berkeley Lab, ALS); P. Yang (UC Berkeley, Berkeley Lab, Kavli Energy Nanoscience Institute); O.M. Yaghi (UC Berkeley, Berkeley Lab, Kavli Energy Nanoscience Institute, King Fahd University of Petroleum and Minerals); C.J. Chang (UC Berkeley, Berkeley Lab, Howard Hughes Medical Institute).
Research funding: U.S. Department of Energy (DOE), Office of Basic Energy Sciences (BES) and Howard Hughes Medical Institute. Operation of the ALS is supported by DOE BES.
Publications about this research: S. Lin, C.S. Diercks, Y.B. Zhang, N. Kornienko, E.M. Nichols, Y. Zhao, A.R. Paris, D. Kim, P. Yang, O.M. Yaghi, and C.J. Chang, “Covalent organic frameworks comprising cobalt porphyrins for catalytic CO2 reduction in water,” Science 349, 1208 (2015); and N. Kornienko, Y. Zhao, C.S. Kley, C. Zhu, D. Kim, S. Lin, C.J. Chang, O.M. Yaghi, and P. Yang, “Metal–Organic Frameworks for Electrocatalytic Reduction of Carbon Dioxide,” J. Am. Chem. Soc. 137, 14129 (2015).
ALS SCIENCE HIGHLIGHT #326