Calcium channel blockers are widely prescribed for heart and blood-vessel diseases. With the help of the high-intensity x-ray beams and remotely controlled robots at the ALS, scientists have revealed, at atomic resolution, how two different classes of calcium channel blocker drugs produce their therapeutic effects. The results pave the way for optimizing these classic compounds for safer and more reliable pharmaceutical applications.
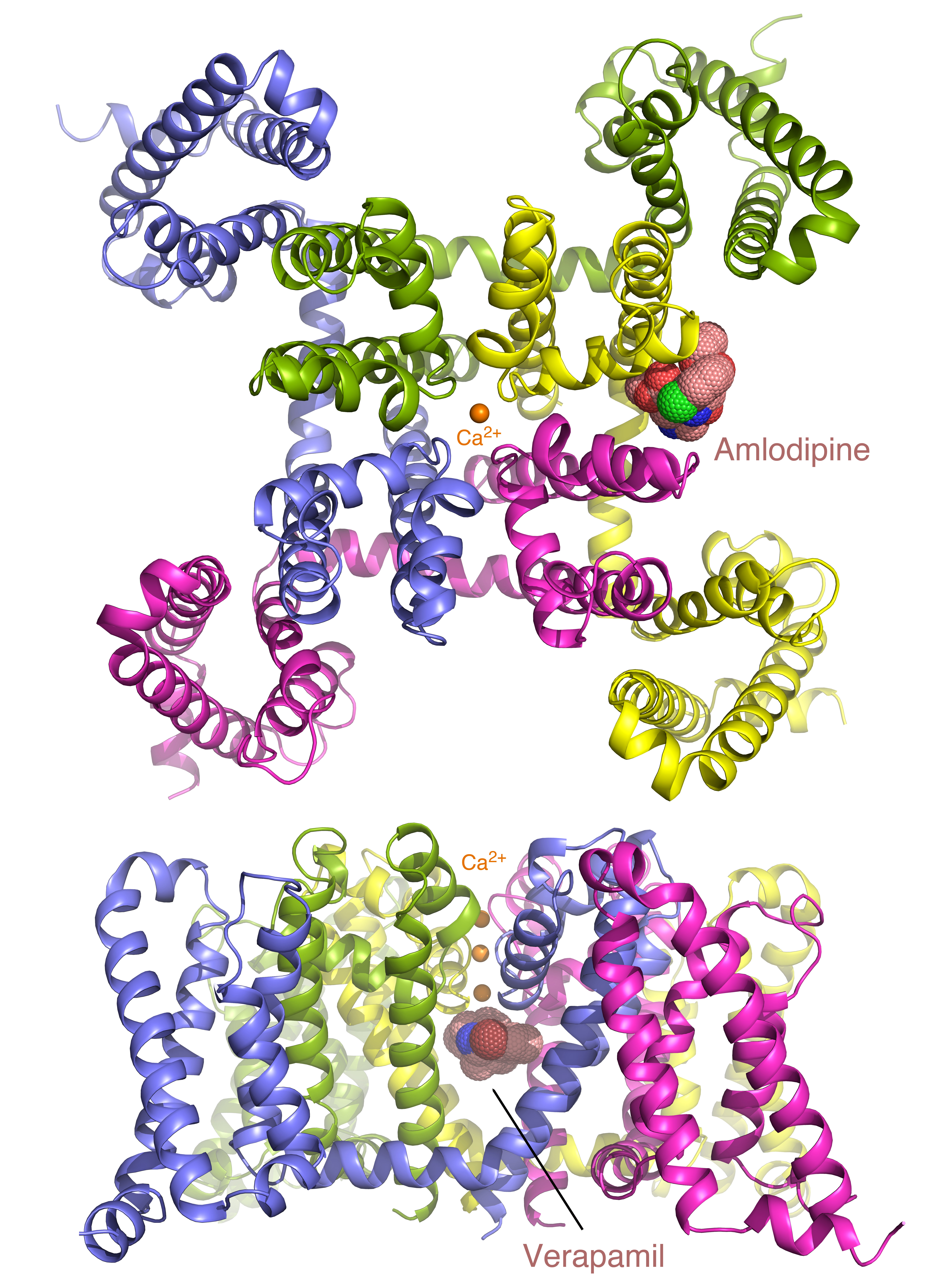
Since the 1960s, three classes of calcium channel blockers have been prescribed for managing a variety of cardiovascular disorders, such as high blood pressure, chest pain, and abnormal heart rhythms. By binding to calcium channel proteins in cell membranes and preventing calcium ions from entering the cell, these drugs can improve contraction rhythm, reduce the workload on the heart, and/or relax blood vessels. Although decades of studies have shed light on the general modes of action of these drugs, the detailed molecular mechanisms by which these drugs work remained unclear. The large size and complexity of human calcium channel proteins make them particularly difficult to characterize using biophysical methods.
Through close collaboration, two research groups at the University of Washington overcame the challenge by first determining the crystal structure of a bacterial ion channel, an ancestor of the human calcium channel but with a much simpler architecture. Using electrophysiological recording methods, these researchers soon realized that two classes of calcium channel blockers, dihydropyridines and phenylalkylamines, effectively block the channel in bacteria as well as in humans. This observation prompted them to use the bacterial channel as a model for analyzing the drugs’ mechanisms of action.
To obtain high-resolution structures of the bacterial channel bound to a drug molecule, the researchers first produced and purified the bacterial channel with each of the two drugs present. Although drug binding did not prevent the channel from being crystallized, many of the crystals were suboptimal for structural analysis by x-ray crystallography. The researchers took advantage of the remotely controllable robot automounter available at the ALS to screen a large number of crystals for the best diffraction. The tunable x-ray source also allowed them to collect anomalous diffraction data at Beamlines 8.2.1 and 8.2.2 with bromine-incorporated drugs, which was critical for locating the drug molecules in the crystal.
After two years of intensive studies, the researchers were able to solve the high-resolution structure of the bacterial channel in complex with a representative member of the two classes of calcium channel blockers. Calcium channels are characterized by a central ion-conducting pore with surrounding voltage sensors that are sensitive to electrical potential. Amlodipine, a drug used for lowering high blood pressure and alleviating chest pain, was found to dock to the outside edge of the channel protein, altering its configuration so that a calcium ion is locked permanently at the entry of the pore. In contrast, verapamil, a drug prescribed to correct abnormal heartbeats, disrupts the ion channel by physically plugging its central cavity and blocking the ion-conduction pathway directly. Because frequent channel opening increases the binding of verapamil to the channel central cavity, the drug inhibits calcium channel function most effectively in rapidly firing cells. This mode of action explains the wide usage of verapamil and similar drugs in treating irregular heart rhythm and reducing heart contraction rate.
By visualizing at high resolution how amlodipine and verapamil differentially bind and act on their target, this study lays the foundation for future rational design of next-generation calcium channel blockers with minimal toxicity and unwanted side effects. The researchers plan to extend their studies to the third class of calcium channel blockers and eventually to human calcium channels for ultimate optimization and fine-tuning of these widely prescribed drugs.
Contact: Ning Zheng
Research conducted by: L. Tang and N. Zheng (Univ. of Washington and Howard Hughes Medical Institute); T.M.Gamal El-Din, T.M. Swanson, T. Scheuer, and W.A. Catterall (Univ. of Washington); and D.C. Pryde (Curadev Pharma, UK).
Research funding: National Institutes of Health and Howard Hughes Medical Institute. Operation of the ALS is supported by the U.S. Department of Energy, Office of Basic Energy Sciences.
Publication about this research: L. Tang, T.M. Gamal El-Din, T.M. Swanson, D.C. Pryde, T. Scheuer, N. Zheng, and W.A. Catterall, “Structural basis for inhibition of a voltage-gated Ca2+ channel by Ca2+ antagonist drugs,” Nature 537, 117 (2016). doi:10.1038/nature19102
ALS SCIENCE HIGHLIGHT #345