SCIENTIFIC ACHIEVEMENT
By encapsulating actinium atoms within a macromolecular complex for analysis using protein crystallography at the Advanced Light Source (ALS), researchers discovered that actinium has a unique solid-state bonding configuration.
SIGNIFICANCE AND IMPACT
A better understanding of actinium behavior could help improve a promising cancer treatment known as targeted alpha therapy.
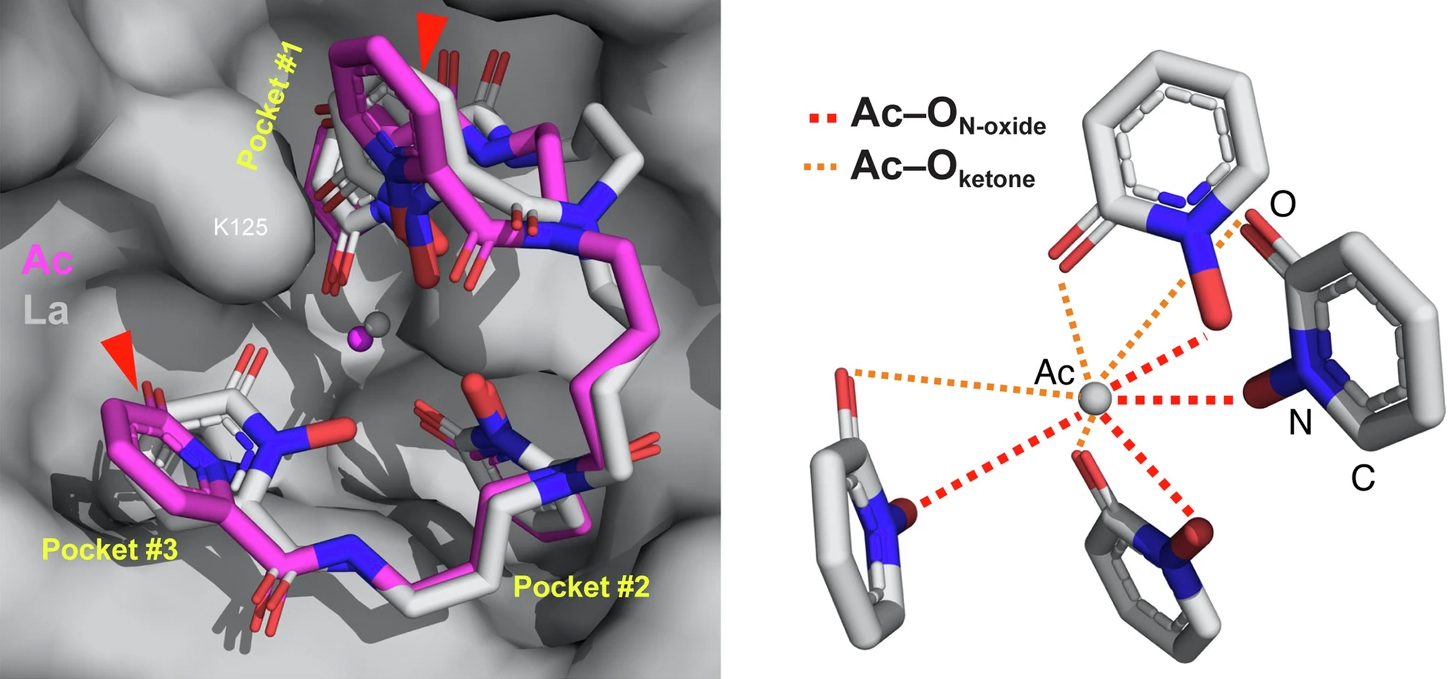
Caught in the actinium
The element actinium (Ac) was first discovered at the turn of the 20th century, but even now, nearly 125 years later, researchers still don’t have a good grasp on the metal’s chemistry. That’s because actinium is only available in extremely small amounts, and working with the radioactive material requires special facilities. But to improve emerging cancer treatments using actinium, researchers will need to better understand how the element binds with other molecules.
In this study, researchers created a macromolecular scaffold, consisting of a synthetic ligand bound to a mammalian protein, to stabilize and “trap” an actinium atom. When crystallized, this macromolecular complex allowed the researchers to probe actinium’s chemical behavior using protein crystallography. While elements often behave similarly to their lighter cousins on the periodic table, researchers were surprised to find that the actinium behaved differently than predicted by similar experiments performed with its lighter counterpart, lanthanum (La).
Targeted alpha therapy
There’s a breadth of applications for elements like actinium, from nuclear energy to medicine to national security. For example, one area of interest is in using an isotope of actinium (actinium-225) in a promising cancer treatment method called targeted alpha therapy (TAT). TAT uses biological delivery systems such as peptides or antibodies to move a radioactive element to the cancer site. When the element decays, it releases energetic alpha particles that travel a short distance, destroying the nearby cancer cells but sparing healthy tissue further away.
Scientists would like to design better delivery systems to get the actinium to particular cells and keep it there, by engineering proteins to bind the actinium with high affinity and either be fused with an antibody or serve as the targeting protein. However, a fundamental understanding of actinium coordination chemistry lags behind the rest of the periodic table because of its limited availability, lack of stable isotopes, and inadequate systems able to probe its chemical behavior. And while nonradioactive lighter elements are often used to predict the chemistry of their radioactive heavier counterparts, in many cases the use of surrogates is not sufficient to understand their chemistry.
Macromolecular scaffold
In this work, researchers bound purified actinium-227 (the longest-lived isotope) to a synthetic molecule (“HOPO”) that is highly effective at binding and removing radioactive elements from inside the body. That bundle was then enveloped inside a mammalian protein, siderocalin (Scn), which has been shown to selectively bind a diverse range of lanthanide and actinide elements. This entire “molecular scaffold” complex was then crystallized, cryocooled, and structurally analyzed using protein crystallography at ALS Beamline 5.0.2. The resulting structure revealed for the first time how actinium interacts with surrounding atoms in the solid state.
The researchers found that the actinium bonding deviated from that of other trivalent metals, such as actinium’s lanthanide surrogate, lanthanum. The comparison captures the limitations of using surrogates in lieu of their radioactive counterparts. In future studies, the researchers will explore actinium-225 (the preferred isotope for targeted alpha therapy) to look for other changes in how the metal binds. They are also interested in pairing actinium with different proteins to learn more about the structures it forms.
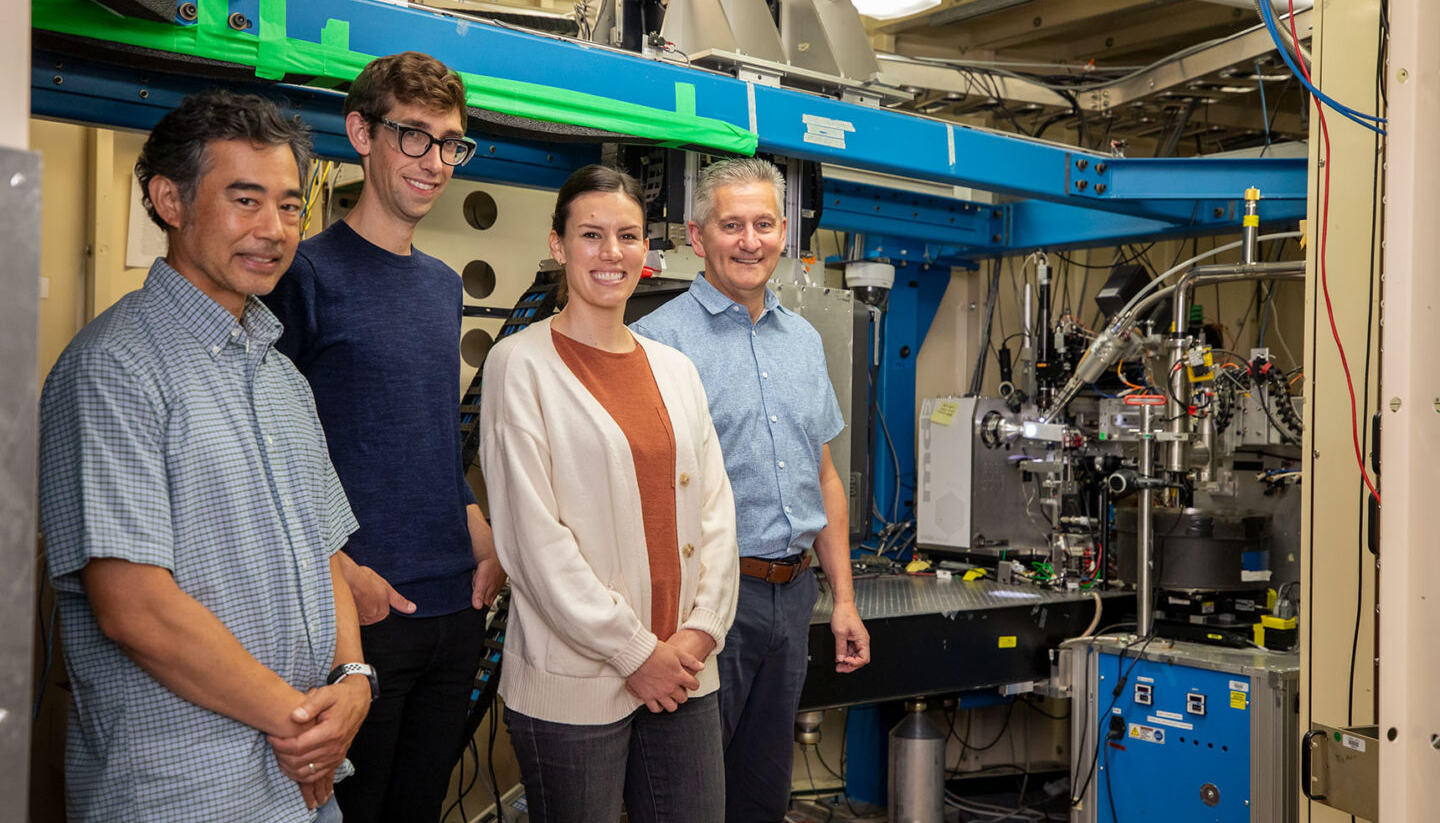
Contact: Rebecca Abergel
Researchers: J.N. Wacker, J.J. Woods, A. Peterson, M. Allaire, W.W. Lukens, and S.G. Minasian (Berkeley Lab); P.B. Rupert and R.K. Strong (Fred Hutchinson Cancer Center); A.N. Gaiser (Berkeley Lab and Michigan State University); and R.J. Abergel (UC Berkeley and Berkeley Lab).
Funding: National Institutes of Health; US Department of Energy, Office of Science, Basic Energy Sciences program (DOE BES), Chemical Sciences, Geosciences, and Biosciences Division. Operation of the ALS is supported by DOE BES.
Publication: J.N. Wacker, J.J. Woods, P.B. Rupert, A. Peterson, M. Allaire, W.W. Lukens, A.N. Gaiser, S.G. Minasian, R.K. Strong, and R.J. Abergel, “Actinium chelation and crystallization in a macromolecular scaffold,” Nat. Commun. 15, 5741 (2024), doi:10.1038/s41467-024-50017-5.
Adapted from the Berkeley Lab news release, “Caught in the Actinium.”
ALS SCIENCE HIGHLIGHT #514